Cell therapy is a kind of medicine aiming to cure disease or alleviate disease symptoms via direct infusion or transplantation of cells, which can be autologous or allogeneic. With several decades’ development and optimization, immuno-oncology cells (such as T cells, nature killer cells, etc.), stem cells (embryonic stem cells, induced pluripotent stem cells, progenitor cells, etc.) or other genetic re-engineered cells have been widely applied for cell therapy. Numerous cell types have been translated into clinical trials and promising cell therapy outcomes have been achieved from Phase I, Phase II and Phase III trials for a great number of diseases.
Landscape of Advanced cell therapy -Index
Immuno-oncology cell therapy
In the past several decades, great progress has been made in the field of anti-tumor therapy, but there are also multiple strategies for tumors to evade the recognition and efficient suppression by the immune system. Therefore, a variety of immunotherapeutic targets (Fig. 1) and strategies have been developed to reactivate and reorganize the human immune system, such as CAR-T, TCR-T, CAR-NK, DC-CIK, oncolytic virus and so on.
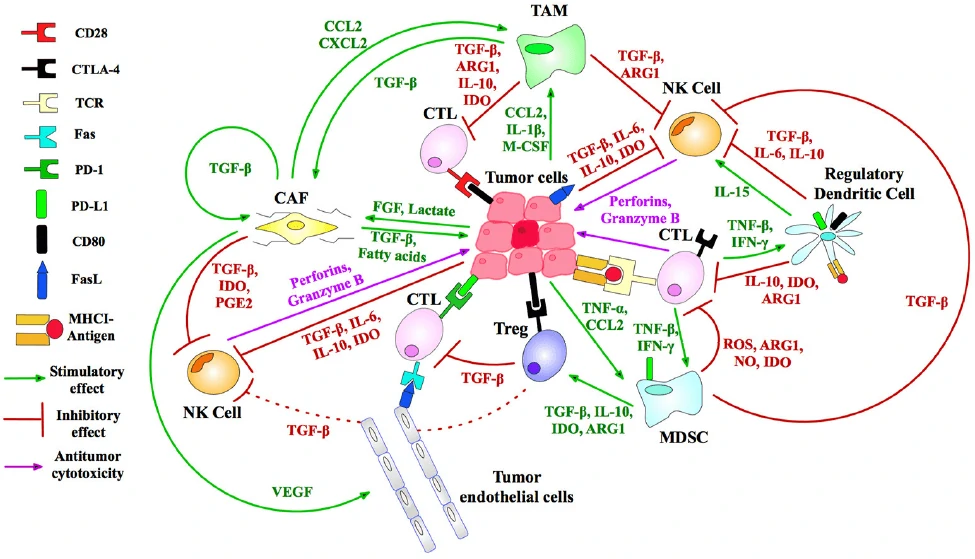
CAR-T
Chimeric antigen receptor (CAR)-modified T cells (CAR-T) are T cells genetically engineered to express CAR (Fig. 2A) [2,3], which can specifically recognize their target antigen via the scFv binding domain of CAR, resulting in T cell activation to specifically target and destroy tumor cells [3-5]. To date, four generations of CAR have been developed according to the structure of the endodomain (Fig. 2B) [3]. Due to little ability to generate enough interleukin-2 (IL-2), the 1st generation CAR-T cells (such as Ag-specific CD3ζ (MFEζ)-CAR-T cells, alpha-folate receptor (FR) -CAR-T cells, CE7R-CAR-T cells, scFv(G250)-CAR-T cells, GD2- CAR-T cells and CD10- CAR-T cells) benefitted substantially from the combination of cytokines [6], and were used for the treatment of different tumors [7-10]. However, most of the studies using the 1st generation CAR-T cells did not show very satisfactory results because of the inadequate proliferation, cytotoxicity, and insufficient secreted cytokines in vivo. To overcome these shortcomings, the 2nd generation CAR-T cells were designed by adding intracellular signaling domains from various co-stimulatory protein receptors to the cytoplasmic tail of the CARs, [11-13]. The 2nd generation CAR-T cells, such as scFvCD19-CD137-CD3-CAR-T cells, MOv19-BBζ-CAR-T cells and scFvCD19-CD28-CD3ζ-CAR-T cells displayed better curative effects on B cell malignancies [12,14]. On the basis of the 2nd generation CAR-T cells, the 3rd generation CAR-T cells were produced with the addition of multiple signaling domains, such as CD3ζ-CD28-OX40 or CD3ζ-CD28-41BB, to promote cytokine production and killing ability. The 3rd generation CAR-T cells, such as CD20-CD28-CD137-CD3ζ-CAR-T cells and HER2-CAR-T cells were applied for the treatment of lymphoma and colon cancer, but with no better outcomes than the 2nd generation CAR-T cells, and the reasons need to be further studied [15,16]. Based on the 2nd generation CAR-T cells, the 4th generation CAR-T cells were obtained with the addition of IL-12, and can mediate T cell redirected for universal cytokine-mediated killing (TRUCKs). TRUCK T cells show great outcomes in disease therapy by augmenting T-cell activation, and also activating and recruiting innate immune cells to destroy the antigen-negative cancer cells in the targeted lesion, and can also be used for the therapy of viral infections, metabolic disorders and auto-immune diseases [17]. Overall, these successive generations of CAR-T cells have yielded remarkable efficacy in several types of cancer or tumor therapy, and some of them have been translated into clinical trials with few side effects (Table 1) [18].
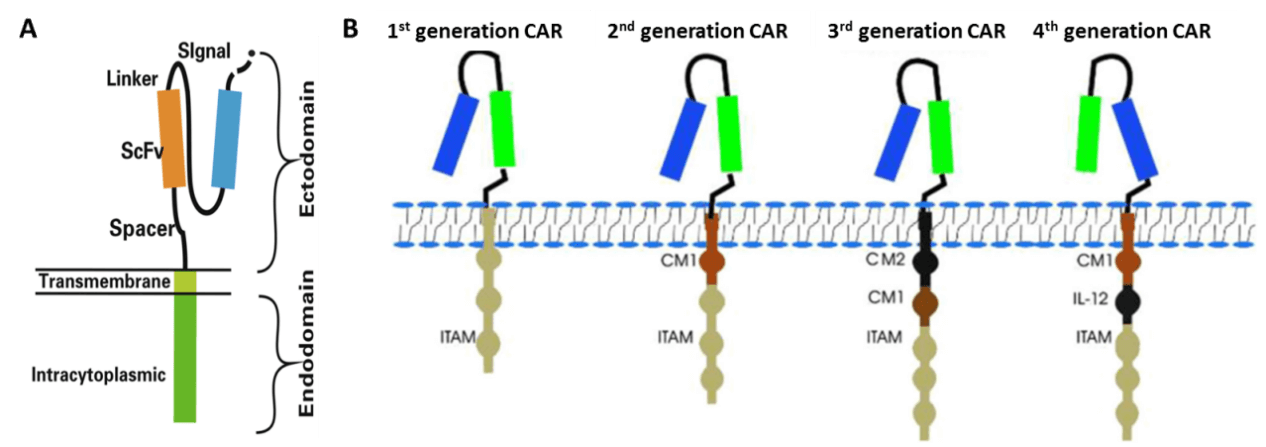
Antigen | Disease | In vitro, in vivo, in preclinical or in clinical trials | NCT/Reference |
CD19 | haematologic malignancies | clinical trials | [19-22] |
CD20 | haematologic malignancies | clinical trials | [23,24] |
TRAIL receptor 1 | Lymphoma | In vitro | [25] |
Kappa | Lymphoma | clinical trials | NCT00881920 |
CD22 | follicular lymphoma, non-Hodgkin’s lymphoma | clinical trials | NCT02315612 |
HA-1 H | Leukaemia | In vitro | [26] |
NKG2D | Leukaemia | clinical trials | NCT02203825 |
FAP | B cell chronic lymphocytic leukaemia | clinical trials | NCT01722149 |
ROR1 | chronic lymphocytic leukaemia | clinical trials | NCT02194374 |
CD138 | multiple myeloma | clinical trials | NCT01886976 |
NY-ESO-1 | multiple myeloma | In vitro | [27] |
Lewis Y | multiple myeloma | clinical trials | NCT01716364 |
HER2 | Osteosarcoma | In vitro | [28] |
HER2 | Breast cancer | In vitro | [29] |
HER2 | Sarcoma | Clinical trial | NCT00902044 |
HER2 | Metastatic cancer | Clinical trial | NCT00924287 |
HER2 | Glioblastoma | Clinical trial | NCT01109095 |
HER2 | Solid tumors | Clinical trial | NCT01935843 |
CEA | Colorectal cancer | In vivo | [30] |
CEA | Colorectal cancer | Clinical trial | NCT00673322 |
CEA | Breast cancer | Clinical trial | NCT00673829 |
CEA | Liver metastases | Clinical trial | NCT01373047 |
CEA | Metastatic cancers | Clinical trial | NCT01723306 |
CSPG4 | Melanoma, breast carcinoma | In vivo | [31] |
EphA2 | Glioblastoma | In vivo | [32] |
FR | Ovarian cancer | In vivo | [33] |
IL-11Rα | Osteosarcoma | In vivo | [34] |
IL-13Rα2 | Glioblastoma | Preclinical trial | [35] |
IL-13Rα2 | Malignant glioma | Clinical trial | NCT02208362 |
IL-13R | Glioma | Preclinical trial | [36] |
CD171 | Neuroblastoma | Clinical trial | NCT02311621 |
EGFR | Advanced EGFR-positive solid tumors | Clinical trial | NCT01869166 |
EGFR | Advanced glioma | Clinical trial | NCT02331693 |
TCR-T
T-cell receptor (TCR)–engineered T-cell therapy (TCR-T) is one potentially powerful treatment that utilizes genetically modified natural T cells to specifically target tumors and destroy tumors with greater potentials [37]. Unlike CARs, TCRs rely on their interactions with peptide-major histocompatibility complex (pMHC), formed by peptide [38,39], generated from intracellular antigen proteolysis, bound with MHC (Fig. 3) [40]. Besides TCR, additional costimulatory or co-inhibitory signals are also required for TCR-T function. For example, CD4 on the surface of helper T cells, which binds to class II MHC complex, and CD8 on the surface of cytotoxic T cells, which binds to class I MHC complex, activates the TCR-T mediated cell destruction [41], while cytotoxic T-lymphocyte antigen 4 (CTLA-4) and programmed cell death protein 1 (PD-1) are responsible for T cell signaling extinguisher [42]. Given that only ~28% antigens are expressed on the cell surface, it is difficult for most of the antigens to be recognized by CAR [43]. Therefore, compared to CAR-T, TCR-T cell therapy shows remarkable advantages over the destruction of tumor cells with intracellular antigens [44]. To date, TCR-T mediated cell therapy has achieved many promising curative potency in both solid tumors and hematological cancers, and some of them have been applied in clinic trials (Table 2).
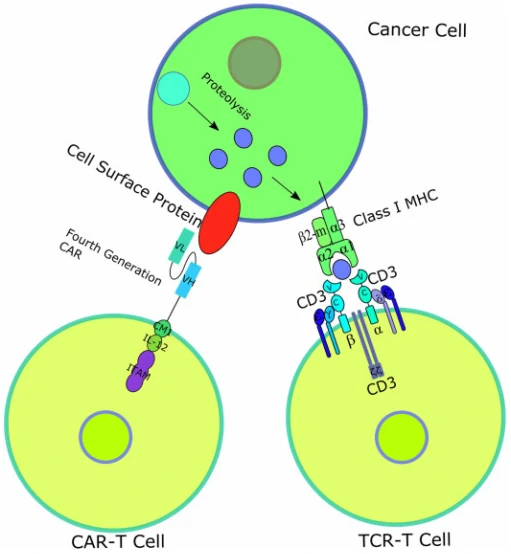
Antigen | Disease | Phase of clinical trials | NCT/Reference |
PRAME | Unknown | Phase I/II | NCT03503968 |
PRAME | Acute myeloid leukemia, myelodysplastic syndrome | Phase I | NCT02743611 |
MAGE-A3/A6 | Unknown | Phase I | NCT03139370 |
Unknown | Head and neck squamous cell carcinoma, squamous cell NSCLC | Phase I | NCT03139370 |
MAGE-A10 | Non-small cell lung carcinoma | Phase I | NCT02592577 |
NY-ESO-1 | Synovial sarcoma | Phase I/II | NCT01343043 |
NY-ESO-1 | Multiple myeloma | Phase I/II | NCT01892293 |
NY-ESO-1 | Multiple myeloma | Phase I/II | NCT01352286 |
NY-ESO-1 | Ovarian cancer | Phase I/II | NCT01567891 |
NY-ESO-1 | Melanoma | Phase I/II | NCT01350401 |
NY-ESO-1 | Non-small cell lung carcinoma | Phase I/II | NCT02588612 |
AFP | Hepatocellular cancer | Phase I | NCT03132792 |
NY-ESO-1 | Refractory multiple myeloma | Phase I | NCT03168438 |
MAGE-A10 | Urinary bladder cancer, head and neck cancer, melanoma | Phase I | NCT02989064 |
CAR-T vs TCR-T
CAR-T and TCR-T both are gene-engineered technologies targeted for the destruction of tumors by improving the recognition and destroy potentials of T cells, thus called “T cell receptor redirection” technologies. However, there are also some differences in the two technologies: 1) CAR-T recognizes their target antigen via the scFv binding domain of CAR, while TCR-T relies on the interactions with peptide-major histocompatibility complex (pMHC) and requires co-stimulatory od co-inhibitory signals; 2) CAR-T recognizes and targets cell surface antigens, while TCR-T can target both cell surface antigens and intracellular antigens; 3) CAR-T shows great therapy outcomes in the treatment of hematological cancers but little effects on solid tumors, while TCR-T displays encouraging curative outcomes in the therapy of solid tumors.
CAR-NK
Although CAR-T has been applied in clinic, quite a lot of side effects, such as off-target, cytokine release syndrome (CRS) etc., pose a great restriction in their further clinical application [47]. Based on natural killer (NK) cells, CAR-NK cell therapy is much safer than CAR-T therapy and becomes a good candidate for targeting and combating solid tumors [48,49]. Based on the four generation of CAR, CARs in CAR-NK are designed with the addition of personalized proteins, such as DNAX-activation protein (DAP) 12 and NKG2 member D (NKG2D), which show great anti-tumor activity in acute myeloid leukemia (ALL), osteosarcoma, and prostate tumors [50-52]. Therefore, CAR-NK cells not only have the ability of CAR to specifically recognize antigen-expressing tumors, but also can destroy tumors via NK cell receptors. To date, numerous CAR-NK cell therapies have been used for anti-tumor therapy in clinical trials (Table 3).
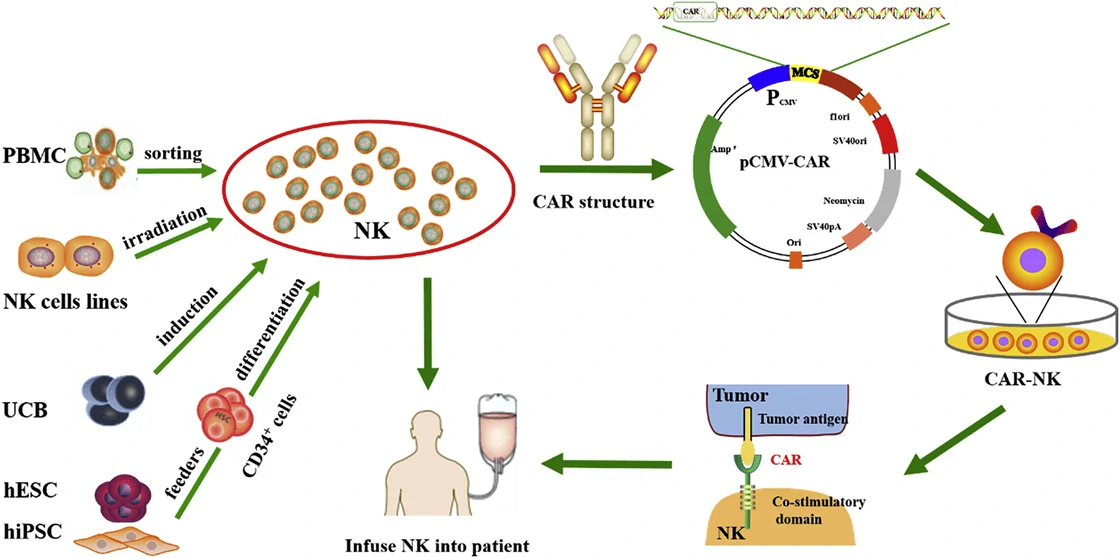
Antigen | Disease | NK source | Phase of clinical trials | NCT/Reference |
CD7 | Lymphoma,leukemia, | NK-92 | Phase I/II | NCT02742727 |
CD19 | Lymphoma,leukemia, | NK-92 | Phase I/II | NCT02892695 |
CD33 | Acute myeloid leukemia | NK-92 | Phase I/II (complete) | NCT02944162 |
MUC1 | Solid tumors | NK-92 | Phase I/II | NCT02839954 |
NR | Non-small cell lung carcinoma | NK-92 | Phase I | NCT03656705 |
HER2 | Glioblastoma multiforme | NK-92 | Phase I | NCT03383978 |
CD19 | B-acute lymphocytic leukaemia | PB-NK | Phase II | NCT01974479 |
CD19 | B-acute lymphocytic leukaemia | PB-NK | Phase I (complete) | NCT00995137 |
CD19 | B-lymphoma | UCB-NK | Phase I/II | NCT03056339 |
Viral vectors used in CAR-T, TCR-T and CAR-NK
The broad application of gene engineered adoptive cell therapy, such as CAR-T, TCR-T and CAR-NK, in clinical trials for the therapy of cancers led to the development of safety-enhanced self-inactivating (SIN) vectors, such as γ-retroviral (gRV), lentiviral (LV) vectors, adenovirus, and AAV vectors. Scalable manufacturing processes for the production of gRV vectors (Fig. 5) and LV vectors using transfection in closed-system bioreactors in compliance with current good manufacturing practices (cGMP) for clinical applications have been developed and optimized [54,55].
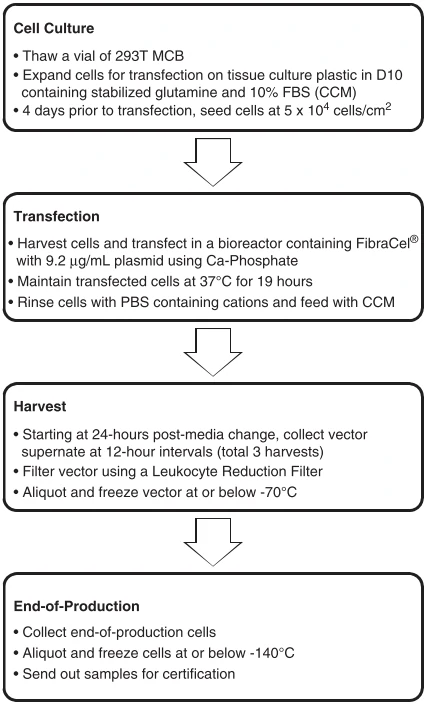
DC-CIK
Cytokine-induced killer cells (CD3+ CD56+ cells, CIK cells) [56] are non-major histocompatibility complex-restricted natural killer T lymphocytes and exhibit stronger anti-tumor and cytolytic activities than lymphokine-activated killer cells [57-59]. Co-cultured with dendritic cells (DCs) or Ag-DC cells, the selective cytotoxicity and anti-tumor immunity of CIK cells would be significantly enhanced, i.e. DC-CIK therapy [60,61]. Compared with traditional treatment, DC-CIK immunotherapy not only improves the clinical indices, but also shows mild adverse and reduces mortality [62].
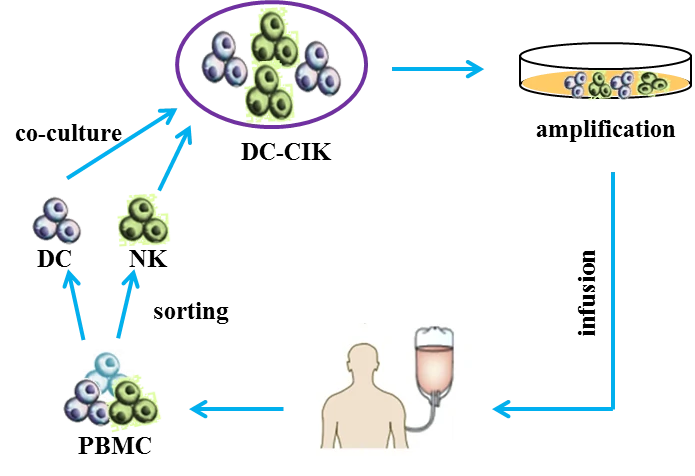
Oncolytic virus
Oncolytic virotherapy is a novel therapeutic modality utilizing oncolytic viruses (such as herpes simplex-, pox-, parvo-, or adenoviruses), which selectively replicate in cancer cells, to directly induce tumor cell lysis or indirectly reactivate human immune system to mediate tumor destruction (Fig. 7) [64]. Through decades’ optimization, a great number of oncolytic virus therapies have been put into clinical trials, such as ONYX-015 (E1B-deleted adenovirus) [65], DNX-2401 (Ad5) [66], CG0070 (Ad5 carrying GM-CSF gene) [67], OBP-301 (Ad5-hTERT-E1A/B) [68], G207 (a conditionally-replicative HSV-1 with ICP34.5 deletion and UL39 disruption) [69], and JX-594 (Pexa-Vec, GM-CSF-enhanced vaccinia virus with TK gene disruption) [67]. Among them, Amgen’s T-VEC (a modified HSV-1 with expression of GM-CSF but deletions in ICP34.5 and ICP47 genes) was the first oncolytic virus approved by America Food and Drug Administration (FDA) for the treatment of melanoma in 2015 [70]. Moreover, when combined with the other therapy, such as Anti-PD-1 immunotherapy, oncolytic virus can significantly promote immune recognition of cancer cell as well as T cell infiltration, and lead to high response rate in patients with advanced melanoma [71].
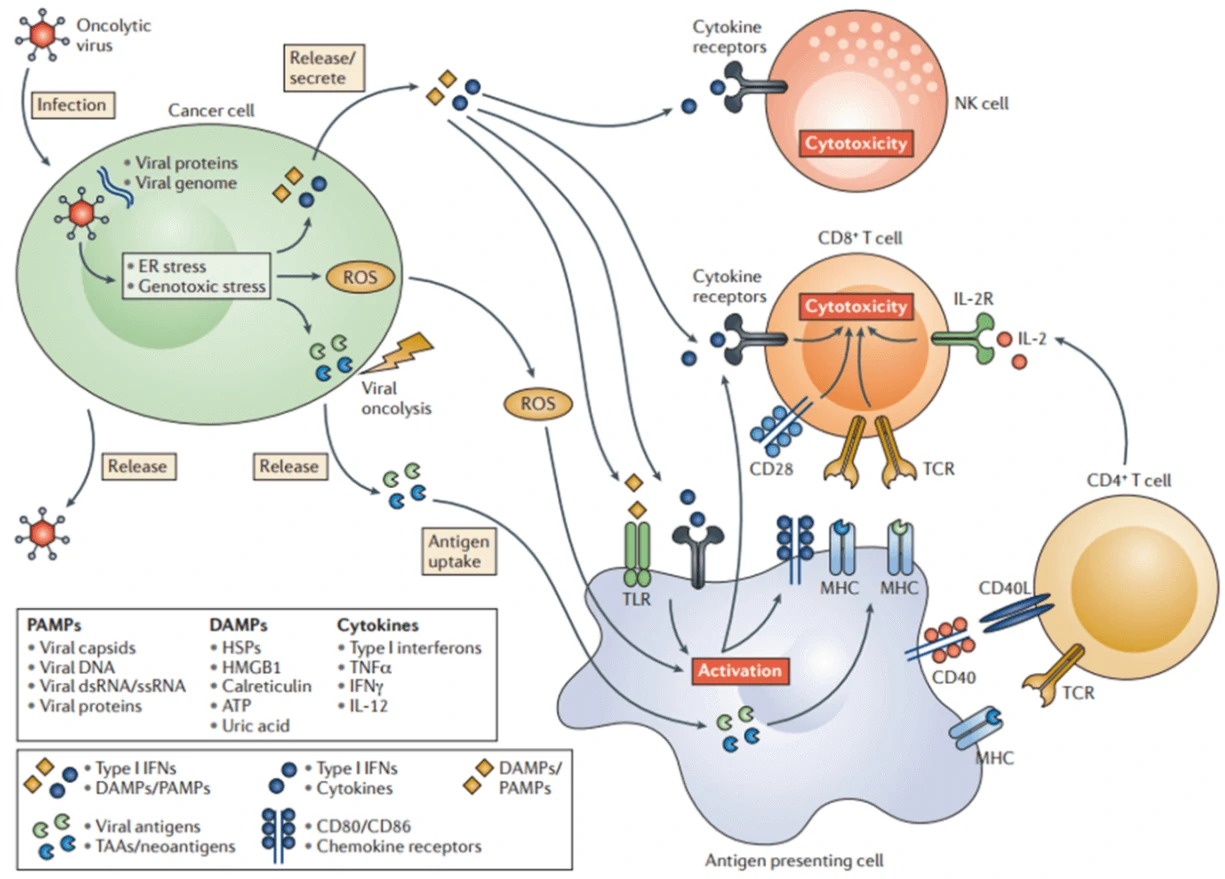
Oncolytic viruses | Disease | Description | NCT/Reference |
Adenovirus | Hepatic carcinoma | Golgi protein 73 (GP73) needed | [72] |
Adenovirus | Pancreatic carcinoma, prostate cancer | Tat-PTD modified hexon and Ad5/35 | [73] |
Adenovirus | Gastric cancer | Acetylcholinesterase (AChE) | [74] |
Adenovirus | Gastric cancer | OBP-301, telomerase-specific | [75] |
Herpes simplex virus 2 | Colon cancer | No virus modification or co-therapies | [76] |
Vaccinia | Colon cancer | Viral Thymidine kinase (TK) deficiency | [77] |
Measles virus | Hepatocellular carcinoma, colon cancer | Retargeted to CD133 | [78] |
Herpes simplex virus | Ovarian cancer | Interleukin−12 | [79] |
ONYX-015 (adenovirus) | Malignant mesothelioma cells | EB1 gene deletion | [65] |
DNX-2401 (adenovirus) | Glioblastoma and gliosarcoma | NCT02197169 | |
CG0070 (adenovirus) | Bladder cancer | carrying CM-CSF gene | NCT02143804, NCT02365818 |
OBP-301 (adenovirus) | carrying hTERT-E1A/B | NCT0229385 | |
HF10 (Herpes simplex virus) | Solid superficial malignant tumors | deletions in neurolatency genes UL43, UL49.5, UL55, and UL56 | NCT02428036 |
G207 (Herpes simplex virus) | Glioblastoma | a conditionally-replicative HSV-1 with ICP34.5 deletion and UL39 disruption | NCT00157703 |
JX-594 (Vaccinia Virus) | Hepatocellular Carcinoma, colorectal cancer | GM-CSF-enhanced vaccinia virus with TK gene disruption | NCT02562755 |
GL-ONC1 (Vaccinia Virus) | Head and neck cancer | TK-inactivated | NCT01443260 |
Reolysin (Retrovirus) | Metastatic melanoma or pancreatic cancer | NCT02514382, NCT02444546 | |
Cavatak™ (Coxsackievirus A21) | Melanoma | Overexpress ICAM-1 and DAF | NCT02565992, NCT02316171 |
Taken together, different kinds of immune-oncology cell therapy show great variety in their curative effects. Although promising therapeutic outcomes in acute lymphocytic leukemia (ALL) and large B cell lymphomas have been achieved by CAR-T [80,81], little therapeutic effects were acquired in solid tumors as well as tumors with intracellular antigens, and multiple side effects, such as cytokine release syndrome, prohibited the broad application of CAR-T [82-84]. TCR-T displays better effects for the treatment of hematopoietic cancers and solid tumors, but requires MHC and co-stimulatory or co-inhibitory signals to combat tumors [45]. Without the drawback of CAR-T, CAR-NK is safer than CAR-T and presents excellent tumor elimination ability in both hematopoietic cancers and solid tumors via CAR-dependent and NK receptor-dependent mechanisms [53]. DC-CIK immunotherapy shows improved immunologic function, reduced mortality, and mild adverse effects in both hematologic malignancies and solid tumors, and may be suitable for patients that are intolerance to radiotherapy and chemotherapy [62,85]. Without the need of defined antigens included in the vectors, oncolytic viruses show a durable anti-tumor effects by directly infecting and lysing tumor cells in situ and also activating the host immune response to eliminate tumor cells, thus displaying curative effects on both hematopoietic cancers [86,87] and solid tumors [64]. Moreover, these immune-oncology cell therapies can also be combined with programmed cell death protein 1 (PD-1) antibody or PD-1 inhibitor, showing reduced drug toxicity and enhanced the tumor cytotoxicity [88]. For example, CD19-CAR-T cell carrying the single-chain variable fragment (scFv) of antibody against PD-1 exhibited potent therapeutic effects superior to those of conventional CAR-T cells [89]; oncolytic Herpes Simplex Virus Type 2 encoding an antibody against PD-1 presented an enhanced therapeutic efficacy with a durable antitumor response both in the tumor microenvironment and in the systemic immune system [90].
Stem Cell Therapy
Cell therapy based on stem cells is a kind of regenerative medicine with great potential for the treatment of diseases via the differentiation ability or the paracrine effects of stem cells as well as their derivatives. To date, a great number of cell types, have been applied from bench to bedside to treat diseases, such as cardiovascular diseases, neurodegenerative diseases and cancers. The cell types can be classified into three generations (Fig. 8): the 1st generation consists of skeletal myoblasts, bone marrow mononuclear cells (BMMNCs) and adipose-derived regenerative cells (ADRCs), mesenchymal stem cells (MSCs) and hematopoietic stem cells (CD34+/CD133+); the 2nd generation mainly utilizes embryonic stem cells (ESCs), induced pluripotent stem cells (iPS), cardiac stem cells (CSC) etc.; the next generation focuses on the combination therapy (CCT) of specific cell types, such as cell mixtures of cardiomyocytes, endothelial cells and smooth muscle cells.
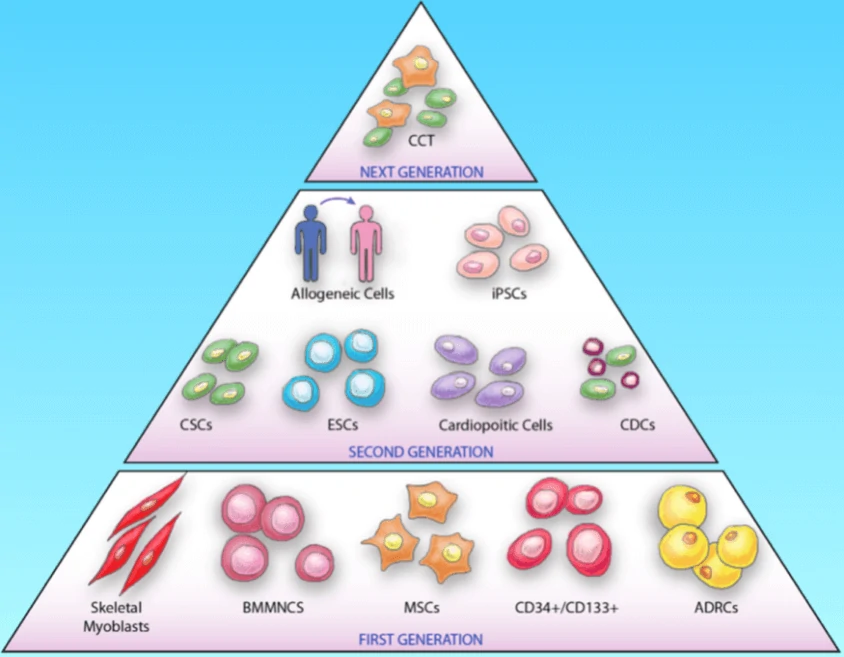
MSC
Mesenchymal stromal/stem cells (MSC) are stromal cells in almost all the tissues or organs, identified by the expression of a specific panel of cell surface markers (CD1051, CD731, CD901, CD34-, CD14-, or CD11b-, CD79- or CD19-, HLA-DR-) and the differentiation potentials into at least osteogenic, adipogenic, and chondrogenic lineages [92]. Although MSCs exist in almost all the human tissues, most of the MSCs used for clinical trials are isolated from bone marrow, adipose tissue and umbilical cord blood. And MSCs may have different biological characteristics and differentiation tendency according to their tissue sources as well as the isolation and culture procedures [93,94]. Based on the strong immunomodulatory, anti-inflammatory, and proregenerative capacities (Fig. 9) [95], MSCs have been promising candidates for the treatment of neurodegenerative diseases, Graft-versus-host disease (GvHD) and so on (Table 5) [96].
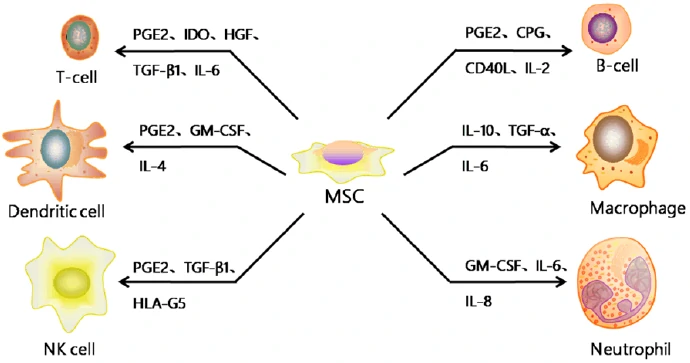
Disease | Delivery route | Clinical trials | Outcome | Reference |
Disease | Delivery route | Clinical trials | Outcome | Reference |
Amyotrophic lateral sclerosis (ALS) | Intrathecally | Phase I | No adverse effects | [97] |
Amyotrophic lateral sclerosis (ALS) | Intramuscularly, intrathecally | Phase I/II | No adverse effects | [98] |
Amyotrophic lateral sclerosis (ALS) | Intrathecally | Phase I | No adverse effects | [99] |
Amyotrophic lateral sclerosis (ALS) | Intrathecally | Phase I/IIa | No adverse effects | [100] |
Multiple sclerosis (MS) | Intravenously | Phase I/II | Intial safety profile and feasibility of the intervention | [101] |
Multiple sclerosis (MS) | Intravenously | Phase I/IIa | Improvement of visual acuity | [102] |
Multiple sclerosis (MS) | Intravenously | Phase I/IIa | Reduction in inflammation | [103] |
ALS/MS | Intrathecally, intravenously | Phase I/II | Induces rapid immunomodulatory effects | [104] |
Spinal cord injury | Intravenously | Phase I | No tumor development | [105] |
Spinal cord injury | Locally injection | Phase III | Variable improvements in tactile sensitivity | [106] |
Spinal cord injury | intramedullary, subdurally | Phase III | Weak therapeutic effect | [107] |
Osteoarthritis | Intraarticulary | Phase I | Improvement of function and pain in high dose group | [108] |
Osteoarthritis | Intraarticulary | Phase I | Improvement in pain levels in low dose group | [109] |
Graft-versus-host disease (GvHD) | Intravenously | Phase I/II | No adverse effects | [110] |
Graft-versus-host disease (GvHD) | Intravenously | Phase I | Immunosuppressive therapy damaged intestinal epithelium | [111] |
Graft-versus-host disease (GvHD) | Intravenously | Phase I/II | Clinical responses | [112] |
Crohn’s disease | Intravenously | Phase I | No adverse effects | [113] |
Crohn’s disease | Intravenously | Phase II | Reduce Crohn’s disease activity index | [114] |
Liver failure | Intravenously | Phase I/II | Reduce Model for End-stage Liver Disease (MELD) score | [115] |
Liver cirrhosis | Intravenously | Phase I | Improvement in MELD score | [116] |
Kidney disease | Intravenously | Phase I | Systemic immunosuppression | [117] |
Acute myocardial infarction | Infarcted sites | Phase I/II | No adverse effects | [118] |
Acute myocardial infarction | Infarct-related artery | Phase II/III | Modest improvement in left ventricular ejection fraction (LVEF) at 6-month follow-up | [119] |
ESCs/iPSCs or their derived cells
With the ability to self-renewal and differentiation into three germ layer derived lineages, embryonic stem cells (ESCs) [120-122] and induced pluripotent stem cells (iPSCs) [123,124] were thought to be invaluable candidates for disease regenerative therapy, especially iPSCs with no or mild immunological rejection (Fig. 10). However, their tumorigenicity greatly limits the direct transplantation of ESCs/iPSCs to treat disease [125]. Then lineage directed progenitor cells, such as neural progenitor/stem cells [126] and cardiac progenitor/stem cells [127], or terminally differentiated cells, such as cardiomyocytes [128,129], endothelial cells [130], smooth muscle cells [131], and retinal pigment epithelium[132] have been widely used in the therapy of a variety of diseases (Table 6).
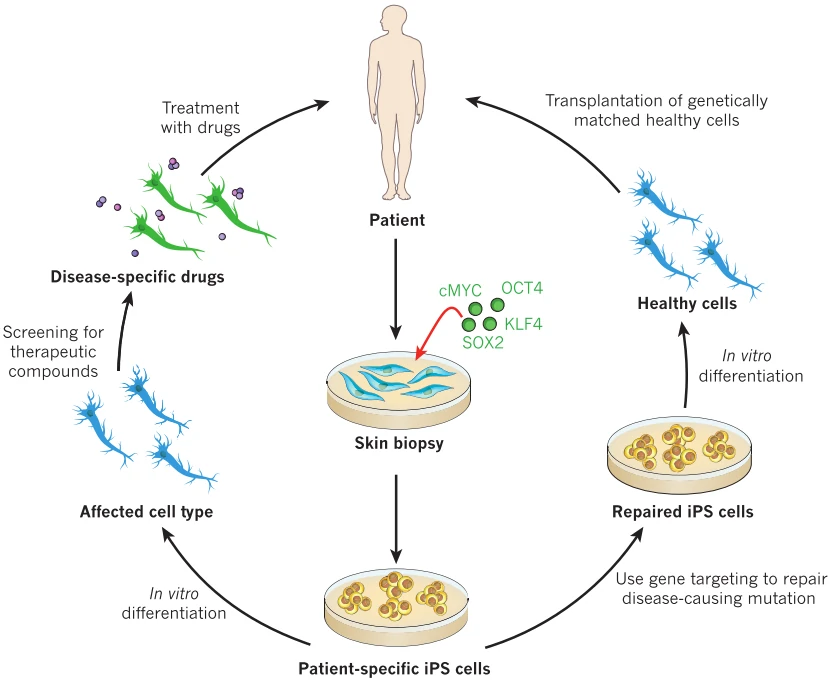
Table 6. Selected examples of disease therapy utilizing Progenitor CellsDiseaseCell typesPhase of clinical trialsDelivery routeOutcomeReferenceIschemic CardiomyopathyCSCsPhase IintracoronaryLVEF increased, scar size reduced, life quality improved[134]Ischemic Cardiomyopathycardiosphere-derived cells (CDCs)Phase IintracoronaryScar size reduced[135]Ischemic CardiomyopathyCardiopoietic cellsPhase IItransendocardial stem cell injectionNo significance[136]Nonischemic CardiomyopathyCD34+ stem cellPhase IIintracoronaryLVEF increased, function capacity improved[137], [138]Macular degenerationhESC-derived retinal pigment epitheliumPhase I/IIsubretinal injectionincrease in subretinal pigmentation, vision-related life quality improved[132], NCT01344993Stargardt’s macular dystrophyhESC-derived retinal pigment epitheliumPhase I/IIsubretinal injectionincrease in subretinal pigmentation, vision-related life quality improved[132], NCT01345006Amyotrophic lateral sclerosis (ALS)neural stem cellsPhase Iintraspinalwell tolerated[139]Amyotrophic lateral sclerosis (ALS)neural stem cellsPhase Iintraspinalwell-tolerated[140]Amyotrophic lateral sclerosis (ALS)neural stem cellsPhase Iintraspinalsafe[141]Parkinson’s diseasehuman parthenogenetic stem cell-derived NSCs (hpNSCs)Phase Istriatumdopamine levels increase[142]Strokeneural stem cellsPhase Istereotactic ipsilateral putamen injectionneurological function improved[143]
Other Cell Therapy
Cell Combination Therapy (CCT) is also a promising therapy with the combination of several cell types, resulting in better therapy outcomes covering the advantages of different cell types. The combination of autologous CSCs and MSCs displayed better cardioreparative effects on swine ischemic cardiomyopathy model than that of MSCs [144,145]. Tri-lineage cell transplantation (cardiomyocytes, endothelial cells, and smooth muscle cells) displayed significant cardiac repair effects in the porcine acute myocardial infarction model by improving left ventricular function, myocardial metabolism, and arteriole density, as well as reducing infarct size and cardiomyocyte apoptosis [146]. Moreover, numerous biocompatible scaffolds, such as hydrogels [147], were used to facilitate the grafts integration in the compromised disease microenvironment and improve the survival of transplanted cells [148], which might significantly promote the clinical application of cell therapy.
Genetic re-engineering cell therapy
Besides the immune-oncology therapy and stem cell derived therapy, gene replacement therapy via lentivirus, adenovirus and adenovirus associated virus (AAV) as well as genetic engineered strategies via CRISPR, ZLN, TALEN have been widely applied in the field of cell therapy [149,150]. For example, PCSK9 gene can be disrupted to prevent cardiovascular disease [151], and PRKAG2 allele (with H530R mutation) can be disrupted to restore the heart morphology and function in Wolff-Parkinson-White syndrome with the wild-type PRKAG2 allele left [152], while pathogenic mutation in the MYBPC3 gene can be corrected in human embryo for the prevention of hypertrophic cardiomyopathy [153]. Besides cardiovascular disease, the mutation in hemoglobin β (HBB) gene was also be seamlessly corrected for the therapy of β-thalassemia [154], and the point mutation in exon 14 of the JAK3 gene in severe combined immunodeficiency (SCID) was corrected to restore the development and maturation ability of T cells [155]. On the basis of the impressive and encouraging outcomes of genetic re-engineering cell therapy, a great number of clinical trials have been carried out to translate the basic scientific achievements into medicines for disease therapy.
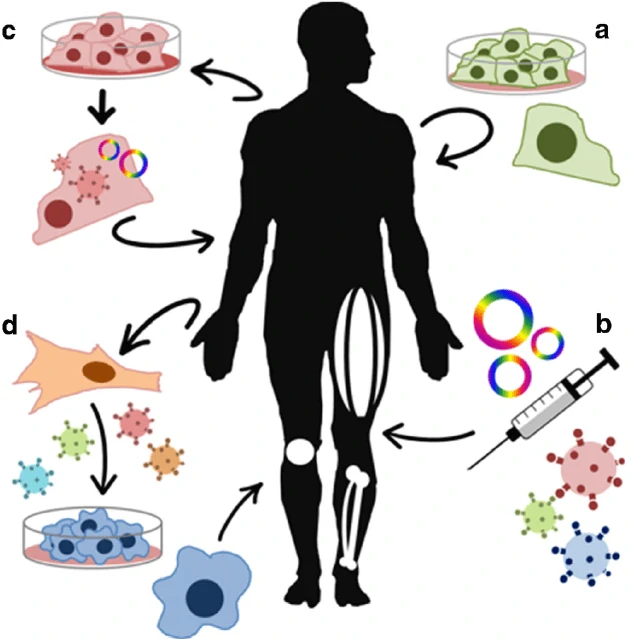
Beta-thalassemia and re-engineering cell therapy
Beta-thalassemia (β-thalassemia), also known as Cooley’s anemia, is a hereditary monogenic hematological disease caused by mutations in the human hemoglobin b (HBB) gene and thus lack of the β-globin chain synthesis [157]. There are two traditional therapeutic methods for the treatment of β-thalassemia: 1) frequent iron chelation therapy, which is easy to cause iron overload and cannot sustain throughout a lifetime [158]; 2) allogeneic hematopoietic stem cell (HSC) transplantation, which is difficult to find a fully-matched donor [159]. Re-engineering cell therapy is a novel therapy without the above drawbacks that utilizes gene editing technology, such as CRISPR, to correct the mutated HBB gene or supplement a functional copy of HBB gene thus restore the synthesis ability of β-globin and cure β-thalassemia (Fig. 12) [160]. Gene therapy by autologous CD34+ cells transduced with the lentiviral BB305 vector, i.e. LentiGlobin, revealed increased hemoglobin levels in patients with no serious adverse effects or no need for long-term red-cell transfusions [161]. To date, LentiGlobin in bluebird bio has been approved to follow the fastest assessment of an advanced therapy medicinal product (ATMP) as part of the European Medicines Agency’s Priority Medicines (PRIME) program.
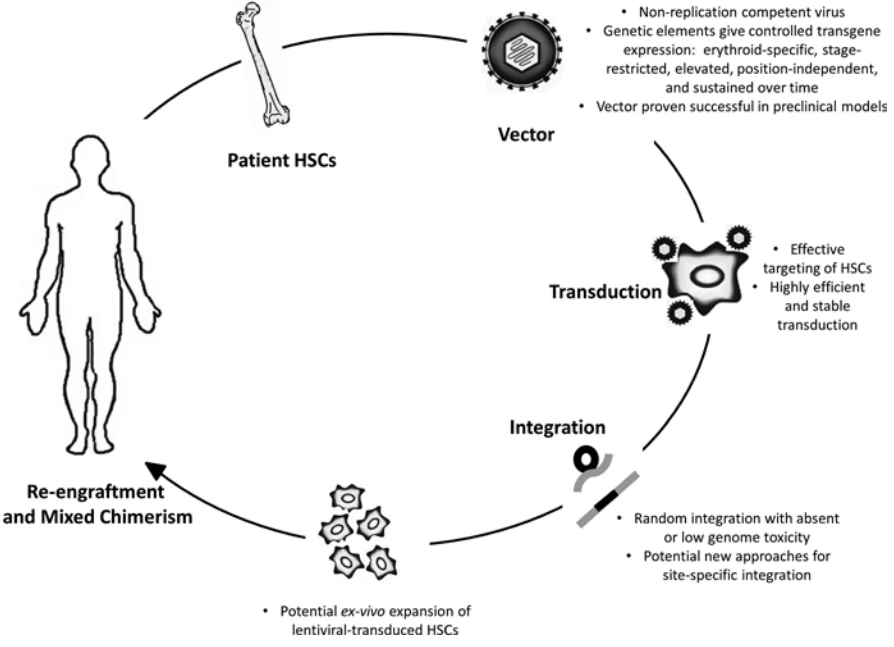
GSK's Strimvelis for ADA-SCID treatment
Adenosine Deaminase (ADA)-Deficient Severe Combined Immune Deficiency (SCID) is a rare, autosomal recessive immunodeficiency, caused by deleterious mutations in ADA gene, for the production of T lymphocytes. There are three therapeutic methods for the treatment of ADA-SCID [162-164]: 1) allogeneic hematopoietic stem cell transplantation, which is difficult to find a fully-matched donor; 2) enzyme replacement therapy (ERT) with polyethylene glycol-conjugated adenosine deaminase, which needs long-term medication as well as high cost (approx. $200,000–400,000 per year) and exhibits great variable availability in different countries [165,166]; 3) autologous HSCT with gene correction of the hematopoietic stem cells, which can avoid the above drawbacks and shows excellent clinical safety and efficacy over the past 15 years [167-170]. In 2016, Strimvelis (also known as GSK2696273, autologous CD34+ cells re-engineered to express ADA) in GlaxoSmithKline was approved by European Commission for the therapy of ADA-SCID.
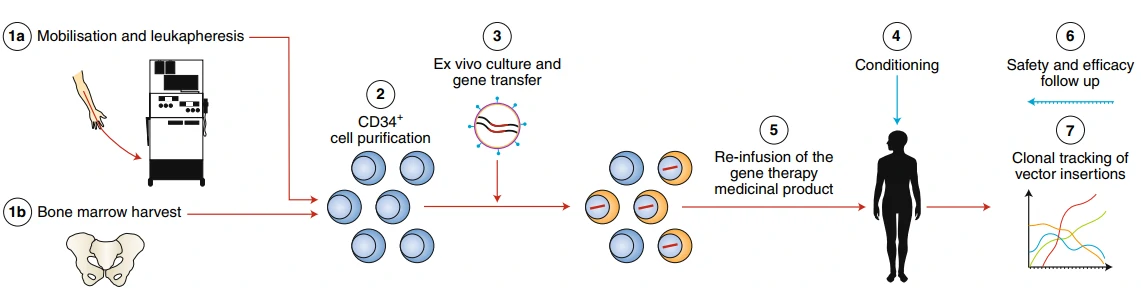
Reference
1.Marshall HT and MBA Djamgoz. (2018). Immuno-Oncology: Emerging Targets and Combination Therapies. Front Oncol 8:315.
2.Eshhar Z, T Waks, G Gross and DG Schindler. (1993). Specific activation and targeting of cytotoxic lymphocytes through chimeric single chains consisting of antibody-binding domains and the gamma or zeta subunits of the immunoglobulin and T-cell receptors. Proc Natl Acad Sci U S A 90:720-4.
3.Zhang C, J Liu, JF Zhong and X Zhang. (2017). Engineering CAR-T cells. Biomark Res 5:22.
4.Curran KJ, HJ Pegram and RJ Brentjens. (2012). Chimeric antigen receptors for T cell immunotherapy: current understanding and future directions. J Gene Med 14:405-15.
5.Dai H, Y Wang, X Lu and W Han. (2016). Chimeric Antigen Receptors Modified T-Cells for Cancer Therapy. J Natl Cancer Inst 108.
6.Brocker T. (2000). Chimeric Fv-zeta or Fv-epsilon receptors are not sufficient to induce activation or cytokine production in peripheral T cells. Blood 96:1999-2001.
7.Bridgeman JS, RE Hawkins, S Bagley, M Blaylock, M Holland and DE Gilham. (2010). The optimal antigen response of chimeric antigen receptors harboring the CD3zeta transmembrane domain is dependent upon incorporation of the receptor into the endogenous TCR/CD3 complex. J Immunol 184:6938-49.
8.Park JR, DL Digiusto, M Slovak, C Wright, A Naranjo, J Wagner, HB Meechoovet, C Bautista, WC Chang, JR Ostberg and MC Jensen. (2007). Adoptive transfer of chimeric antigen receptor re-directed cytolytic T lymphocyte clones in patients with neuroblastoma. Mol Ther 15:825-33.
9.Kershaw MH, JA Westwood, LL Parker, G Wang, Z Eshhar, SA Mavroukakis, DE White, JR Wunderlich, S Canevari, L Rogers-Freezer, CC Chen, JC Yang, SA Rosenberg and P Hwu. (2006). A phase I study on adoptive immunotherapy using gene-modified T cells for ovarian cancer. Clin Cancer Res 12:6106-15.
10.Lamers CH, SC Langeveld, CM Groot-van Ruijven, R Debets, S Sleijfer and JW Gratama. (2007). Gene-modified T cells for adoptive immunotherapy of renal cell cancer maintain transgene-specific immune functions in vivo. Cancer Immunol Immunother 56:1875-83.
11.Finney HM, AD Lawson, CR Bebbington and AN Weir. (1998). Chimeric receptors providing both primary and costimulatory signaling in T cells from a single gene product. J Immunol 161:2791-7.
12.Dotti G, B Savoldo and M Brenner. (2009). Fifteen years of gene therapy based on chimeric antigen receptors: “are we nearly there yet?”. Hum Gene Ther 20:1229-39.
13.Park TS, SA Rosenberg and RA Morgan. (2011). Treating cancer with genetically engineered T cells. Trends Biotechnol 29:550-7.
14.Cai B, M Guo, Y Wang, Y Zhang, J Yang, Y Guo, H Dai, C Yu, Q Sun, J Qiao, K Hu, H Zuo, Z Dong, Z Zhang, M Feng, B Li, Y Sun, T Liu, Z Liu, Y Wang, Y Huang, B Yao, W Han and H Ai. (2016). Co-infusion of haplo-identical CD19-chimeric antigen receptor T cells and stem cells achieved full donor engraftment in refractory acute lymphoblastic leukemia. J Hematol Oncol 9:131.
15.Till BG, MC Jensen, J Wang, X Qian, AK Gopal, DG Maloney, CG Lindgren, Y Lin, JM Pagel, LE Budde, A Raubitschek, SJ Forman, PD Greenberg, SR Riddell and OW Press. (2012). CD20-specific adoptive immunotherapy for lymphoma using a chimeric antigen receptor with both CD28 and 4-1BB domains: pilot clinical trial results. Blood 119:3940-50.
16.Morgan RA, JC Yang, M Kitano, ME Dudley, CM Laurencot and SA Rosenberg. (2010). Case report of a serious adverse event following the administration of T cells transduced with a chimeric antigen receptor recognizing ERBB2. Mol Ther 18:843-51.
17.Chmielewski M and H Abken. (2015). TRUCKs: the fourth generation of CARs. Expert Opin Biol Ther 15:1145-54.
18.Sha HH, DD Wang, DL Yan, Y Hu, SJ Yang, SW Liu and JF Feng. (2017). Chimaeric antigen receptor T-cell therapy for tumour immunotherapy. Biosci Rep 37.
19.Savoldo B, CA Ramos, E Liu, MP Mims, MJ Keating, G Carrum, RT Kamble, CM Bollard, AP Gee, Z Mei, H Liu, B Grilley, CM Rooney, HE Heslop, MK Brenner and G Dotti. (2011). CD28 costimulation improves expansion and persistence of chimeric antigen receptor-modified T cells in lymphoma patients. J Clin Invest 121:1822-6.
20.Kochenderfer JN, ME Dudley, SA Feldman, WH Wilson, DE Spaner, I Maric, M Stetler-Stevenson, GQ Phan, MS Hughes, RM Sherry, JC Yang, US Kammula, L Devillier, R Carpenter, DA Nathan, RA Morgan, C Laurencot and SA Rosenberg. (2012). B-cell depletion and remissions of malignancy along with cytokine-associated toxicity in a clinical trial of anti-CD19 chimeric-antigen-receptor-transduced T cells. Blood 119:2709-20.
21.Brentjens RJ, I Riviere, JH Park, ML Davila, X Wang, J Stefanski, C Taylor, R Yeh, S Bartido, O Borquez-Ojeda, M Olszewska, Y Bernal, H Pegram, M Przybylowski, D Hollyman, Y Usachenko, D Pirraglia, J Hosey, E Santos, E Halton, P Maslak, D Scheinberg, J Jurcic, M Heaney, G Heller, M Frattini and M Sadelain. (2011). Safety and persistence of adoptively transferred autologous CD19-targeted T cells in patients with relapsed or chemotherapy refractory B-cell leukemias. Blood 118:4817-28.
22.Porter DL, BL Levine, M Kalos, A Bagg and CH June. (2011). Chimeric antigen receptor-modified T cells in chronic lymphoid leukemia. N Engl J Med 365:725-33.
23.Till BG, MC Jensen, J Wang, EY Chen, BL Wood, HA Greisman, X Qian, SE James, A Raubitschek, SJ Forman, AK Gopal, JM Pagel, CG Lindgren, PD Greenberg, SR Riddell and OW Press. (2008). Adoptive immunotherapy for indolent non-Hodgkin lymphoma and mantle cell lymphoma using genetically modified autologous CD20-specific T cells. Blood 112:2261-71.
24.Wang Y, WY Zhang, QW Han, Y Liu, HR Dai, YL Guo, J Bo, H Fan, Y Zhang, YJ Zhang, MX Chen, KC Feng, QS Wang, XB Fu and WD Han. (2014). Effective response and delayed toxicities of refractory advanced diffuse large B-cell lymphoma treated by CD20-directed chimeric antigen receptor-modified T cells. Clin Immunol 155:160-75.
25.Kobayashi E, H Kishi, T Ozawa, H Hamana, H Nakagawa, A Jin, Z Lin and A Muraguchi. (2014). A chimeric antigen receptor for TRAIL-receptor 1 induces apoptosis in various types of tumor cells. Biochem Biophys Res Commun 453:798-803.
26.Inaguma Y, Y Akahori, Y Murayama, K Shiraishi, S Tsuzuki-Iba, A Endoh, J Tsujikawa, A Demachi-Okamura, K Hiramatsu, H Saji, Y Yamamoto, N Yamamoto, Y Nishimura, T Takahashi, K Kuzushima, N Emi and Y Akatsuka. (2014). Construction and molecular characterization of a T-cell receptor-like antibody and CAR-T cells specific for minor histocompatibility antigen HA-1H. Gene Ther 21:575-84.
27.Schuberth PC, G Jakka, SM Jensen, A Wadle, F Gautschi, D Haley, S Haile, A Mischo, G Held, M Thiel, M Tinguely, CB Bifulco, BA Fox, C Renner and U Petrausch. (2013). Effector memory and central memory NY-ESO-1-specific re-directed T cells for treatment of multiple myeloma. Gene Ther 20:386-95.
28.Mata M, JF Vera, C Gerken, CM Rooney, T Miller, C Pfent, LL Wang, HM Wilson-Robles and S Gottschalk. (2014). Toward immunotherapy with redirected T cells in a large animal model: ex vivo activation, expansion, and genetic modification of canine T cells. J Immunother 37:407-15.
29.Hu WX, HP Chen, K Yu, LX Shen, CY Wang, SZ Su, WJ Sui, DM Shan and HZ Li. (2012). Gene therapy of malignant solid tumors by targeting erbB2 receptors and by activating T cells. Cancer Biother Radiopharm 27:711-8.
30.Blat D, E Zigmond, Z Alteber, T Waks and Z Eshhar. (2014). Suppression of murine colitis and its associated cancer by carcinoembryonic antigen-specific regulatory T cells. Mol Ther 22:1018-28.
31.Geldres C, B Savoldo, V Hoyos, I Caruana, M Zhang, E Yvon, M Del Vecchio, CJ Creighton, M Ittmann, S Ferrone and G Dotti. (2014). T lymphocytes redirected against the chondroitin sulfate proteoglycan-4 control the growth of multiple solid tumors both in vitro and in vivo. Clin Cancer Res 20:962-71.
32.Chow KK, S Naik, S Kakarla, VS Brawley, DR Shaffer, Z Yi, N Rainusso, MF Wu, H Liu, Y Kew, RG Grossman, S Powell, D Lee, N Ahmed and S Gottschalk. (2013). T cells redirected to EphA2 for the immunotherapy of glioblastoma. Mol Ther 21:629-37.
33.Lanitis E, M Poussin, IS Hagemann, G Coukos, R Sandaltzopoulos, N Scholler and DJ Powell, Jr. (2012). Redirected antitumor activity of primary human lymphocytes transduced with a fully human anti-mesothelin chimeric receptor. Mol Ther 20:633-43.
34.Huang G, L Yu, LJ Cooper, M Hollomon, H Huls and ES Kleinerman. (2012). Genetically modified T cells targeting interleukin-11 receptor alpha-chain kill human osteosarcoma cells and induce the regression of established osteosarcoma lung metastases. Cancer Res 72:271-81.
35.Krebs S, KK Chow, Z Yi, T Rodriguez-Cruz, M Hegde, C Gerken, N Ahmed and S Gottschalk. (2014). T cells redirected to interleukin-13Ralpha2 with interleukin-13 mutein–chimeric antigen receptors have anti-glioma activity but also recognize interleukin-13Ralpha1. Cytotherapy 16:1121-31.
36.Kong S, S Sengupta, B Tyler, AJ Bais, Q Ma, S Doucette, J Zhou, A Sahin, BS Carter, H Brem, RP Junghans and P Sampath. (2012). Suppression of human glioma xenografts with second-generation IL13R-specific chimeric antigen receptor-modified T cells. Clin Cancer Res 18:5949-60.
37.Mo Z, P Du, G Wang and Y Wang. (2017). The Multi-Purpose Tool of Tumor Immunotherapy: Gene-Engineered T Cells. J Cancer 8:1690-1703.
38.Hewitt EW. (2003). The MHC class I antigen presentation pathway: strategies for viral immune evasion. Immunology 110:163-9.
39.Buonaguro L, A Petrizzo, ML Tornesello and FM Buonaguro. (2011). Translating tumor antigens into cancer vaccines. Clin Vaccine Immunol 18:23-34.
40.Kass I, AM Buckle and NA Borg. (2014). Understanding the structural dynamics of TCR-pMHC complex interactions. Trends Immunol 35:604-612.
41.Wieczorek M, ET Abualrous, J Sticht, M Alvaro-Benito, S Stolzenberg, F Noe and C Freund. (2017). Major Histocompatibility Complex (MHC) Class I and MHC Class II Proteins: Conformational Plasticity in Antigen Presentation. Front Immunol 8:292.
42.Pardoll DM. (2012). The blockade of immune checkpoints in cancer immunotherapy. Nat Rev Cancer 12:252-64.
43.Uhlen M, L Fagerberg, BM Hallstrom, C Lindskog, P Oksvold, A Mardinoglu, A Sivertsson, C Kampf, E Sjostedt, A Asplund, I Olsson, K Edlund, E Lundberg, S Navani, CA Szigyarto, J Odeberg, D Djureinovic, JO Takanen, S Hober, T Alm, PH Edqvist, H Berling, H Tegel, J Mulder, J Rockberg, P Nilsson, JM Schwenk, M Hamsten, K von Feilitzen, M Forsberg, L Persson, F Johansson, M Zwahlen, G von Heijne, J Nielsen and F Ponten. (2015). Proteomics. Tissue-based map of the human proteome. Science 347:1260419.
44.Harris DT and DM Kranz. (2016). Adoptive T Cell Therapies: A Comparison of T Cell Receptors and Chimeric Antigen Receptors. Trends Pharmacol Sci 37:220-230.
45.Zhang J and L Wang. (2019). The Emerging World of TCR-T Cell Trials Against Cancer: A Systematic Review. Technol Cancer Res Treat 18:1533033819831068.
46.Golubovskaya V, R Berahovich, S Xu, H Harto and L Wu. (2017). Major Highlights of the CAR-TCR Summit, Boston, 2016. Anticancer Agents Med Chem 17:1344-1350.
47.Chow VA, AK Gopal, DG Maloney, CJ Turtle, SD Smith, CS Ujjani, M Shadman, RD Cassaday, BG Till, YD Tseng, EH Warren, AR Shustov, MP Menon, S Bhark, UH Acharya, E Mullane, LM Hannan, JM Voutsinas, TA Gooley and RC Lynch. (2019). Outcomes of patients with large B-cell lymphomas and progressive disease following CD19-specific CAR T-cell therapy. Am J Hematol 94:E209-E213.
48.Zhang C, P Oberoi, S Oelsner, A Waldmann, A Lindner, T Tonn and WS Wels. (2017). Chimeric Antigen Receptor-Engineered NK-92 Cells: An Off-the-Shelf Cellular Therapeutic for Targeted Elimination of Cancer Cells and Induction of Protective Antitumor Immunity. Front Immunol 8:533.
49.Barry KC, J Hsu, ML Broz, FJ Cueto, M Binnewies, AJ Combes, AE Nelson, K Loo, R Kumar, MD Rosenblum, MD Alvarado, DM Wolf, D Bogunovic, N Bhardwaj, AI Daud, PK Ha, WR Ryan, JL Pollack, B Samad, S Asthana, V Chan and MF Krummel. (2018). A natural killer-dendritic cell axis defines checkpoint therapy-responsive tumor microenvironments. Nat Med 24:1178-1191.
50.Gilfillan S, EL Ho, M Cella, WM Yokoyama and M Colonna. (2002). NKG2D recruits two distinct adapters to trigger NK cell activation and costimulation. Nat Immunol 3:1150-5.
51.Upshaw JL, LN Arneson, RA Schoon, CJ Dick, DD Billadeau and PJ Leibson. (2006). NKG2D-mediated signaling requires a DAP10-bound Grb2-Vav1 intermediate and phosphatidylinositol-3-kinase in human natural killer cells. Nat Immunol 7:524-32.
52.Chang YH, J Connolly, N Shimasaki, K Mimura, K Kono and D Campana. (2013). A chimeric receptor with NKG2D specificity enhances natural killer cell activation and killing of tumor cells. Cancer Res 73:1777-86.
53.Wang W, J Jiang and C Wu. (2019). CAR-NK for tumor immunotherapy: Clinical transformation and future prospects. Cancer Lett.
54.Lemiale F, B Asefa, D Ye, C Chen, N Korokhov and L Humeau. (2010). An HIV-based lentiviral vector as HIV vaccine candidate: Immunogenic characterization. Vaccine 28:1952-61.
55.van der Loo JC, WP Swaney, E Grassman, A Terwilliger, T Higashimoto, A Schambach, C Baum, AJ Thrasher, DA Williams, DL Nordling, L Reeves and P Malik. (2012). Scale-up and manufacturing of clinical-grade self-inactivating gamma-retroviral vectors by transient transfection. Gene Ther 19:246-54.
56.Schmidt-Wolf IG, RS Negrin, HP Kiem, KG Blume and IL Weissman. (1991). Use of a SCID mouse/human lymphoma model to evaluate cytokine-induced killer cells with potent antitumor cell activity. J Exp Med 174:139-49.
57.Linn YC, LC Lau and KM Hui. (2002). Generation of cytokine-induced killer cells from leukaemic samples with in vitro cytotoxicity against autologous and allogeneic leukaemic blasts. Br J Haematol 116:78-86.
58.Li DP, W Li, J Feng, K Chen and M Tao. (2015). Adjuvant chemotherapy with sequential cytokine-induced killer (CIK) cells in stage IB non-small cell lung cancer. Oncol Res 22:67-74.
59.Liu P, L Chen and X Huang. (2009). The antitumor effects of CIK cells combined with docetaxel against drug-resistant lung adenocarcinoma cell line SPC-A1/DTX in vitro and in vivo. Cancer Biother Radiopharm 24:91-8.
60.Zhang S, SJ Jiang, CQ Zhang, HM Wang and CX Bai. (2005). Antitumour activities of cytokine-induced killer cells and dendritic cells in vitro and in vivo. Chin Med J (Engl) 118:1308-12.
61.Wang YF, PE Kunda, JW Lin, H Wang, XM Chen, QL Liu and T Liu. (2013). Cytokine-induced killer cells co-cultured with complete tumor antigen-loaded dendritic cells, have enhanced selective cytotoxicity on carboplatin-resistant retinoblastoma cells. Oncol Rep 29:1841-50.
62.Liu YL, LX Yang, F Zhang, BS Tang, LT Zhao, JR Zhu, QY Jin, RX Wang and YM Li. (2019). Clinical effect and safety of dendritic cell-cytokine-induced killer cell immunotherapy for pancreatic cancer: a systematic review and meta-analysis. Cytotherapy 21:1064-1080.
63.Zhang J, H Li, D Gao, B Zhang, M Zheng, M Lun, M Wei, R Duan, M Guo, J Hua, Q Liu, J Bai, H Liu, J Zheng and H Yao. (2018). A prognosis and impact factor analysis of DC-CIK cell therapy for patients with hepatocellular carcinoma undergoing postoperative TACE. Cancer Biol Ther 19:475-483.
64.Kaufman HL, FJ Kohlhapp and A Zloza. (2015). Oncolytic viruses: a new class of immunotherapy drugs. Nat Rev Drug Discov 14:642-62.
65.Garber K. (2006). China approves world’s first oncolytic virus therapy for cancer treatment. J Natl Cancer Inst 98:298-300.
66.Kaufman HL, FJ Kohlhapp and A Zloza. (2016). Oncolytic viruses: a new class of immunotherapy drugs. Nat Rev Drug Discov 15:660.
67.Heo J, T Reid, L Ruo, CJ Breitbach, S Rose, M Bloomston, M Cho, HY Lim, HC Chung, CW Kim, J Burke, R Lencioni, T Hickman, A Moon, YS Lee, MK Kim, M Daneshmand, K Dubois, L Longpre, M Ngo, C Rooney, JC Bell, BG Rhee, R Patt, TH Hwang and DH Kirn. (2013). Randomized dose-finding clinical trial of oncolytic immunotherapeutic vaccinia JX-594 in liver cancer. Nat Med 19:329-36.
68.Kawashima T, S Kagawa, N Kobayashi, Y Shirakiya, T Umeoka, F Teraishi, M Taki, S Kyo, N Tanaka and T Fujiwara. (2004). Telomerase-specific replication-selective virotherapy for human cancer. Clin Cancer Res 10:285-92.
69.Markert JM, SN Razdan, HC Kuo, A Cantor, A Knoll, M Karrasch, LB Nabors, M Markiewicz, BS Agee, JM Coleman, AD Lakeman, CA Palmer, JN Parker, RJ Whitley, RR Weichselbaum, JB Fiveash and GY Gillespie. (2014). A phase 1 trial of oncolytic HSV-1, G207, given in combination with radiation for recurrent GBM demonstrates safety and radiographic responses. Mol Ther 22:1048-55.
70.Andtbacka RH, HL Kaufman, F Collichio, T Amatruda, N Senzer, J Chesney, KA Delman, LE Spitler, I Puzanov, SS Agarwala, M Milhem, L Cranmer, B Curti, K Lewis, M Ross, T Guthrie, GP Linette, GA Daniels, K Harrington, MR Middleton, WH Miller, Jr., JS Zager, Y Ye, B Yao, A Li, S Doleman, A VanderWalde, J Gansert and RS Coffin. (2015). Talimogene Laherparepvec Improves Durable Response Rate in Patients With Advanced Melanoma. J Clin Oncol 33:2780-8.
71.Ribas A, R Dummer, I Puzanov, A VanderWalde, RHI Andtbacka, O Michielin, AJ Olszanski, J Malvehy, J Cebon, E Fernandez, JM Kirkwood, TF Gajewski, L Chen, KS Gorski, AA Anderson, SJ Diede, ME Lassman, J Gansert, FS Hodi and GV Long. (2017). Oncolytic Virotherapy Promotes Intratumoral T Cell Infiltration and Improves Anti-PD-1 Immunotherapy. Cell 170:1109-1119 e10.
72.Zhang X, S Meng, R Zhang, B Ma, T Liu, Y Yang, W Xie, X Liu, F Huang, T Liu, X Zhou, X Liu and Y Wang. (2016). GP73-regulated oncolytic adenoviruses possess potent killing effect on human liver cancer stem-like cells. Oncotarget 7:29346-58.
73.Yu D, C Jin, M Ramachandran, J Xu, B Nilsson, O Korsgren, K Le Blanc, L Uhrbom, K Forsberg-Nilsson, B Westermark, R Adamson, N Maitland, X Fan and M Essand. (2013). Adenovirus serotype 5 vectors with Tat-PTD modified hexon and serotype 35 fiber show greatly enhanced transduction capacity of primary cell cultures. PLoS One 8:e54952.
74.Xu H, Z Shen, J Xiao, Y Yang, W Huang, Z Zhou, J Shen, Y Zhu, XY Liu and L Chu. (2014). Acetylcholinesterase overexpression mediated by oncolytic adenovirus exhibited potent anti-tumor effect. BMC Cancer 14:668.
75.Yano S, H Tazawa, Y Hashimoto, Y Shirakawa, S Kuroda, M Nishizaki, H Kishimoto, F Uno, T Nagasaka, Y Urata, S Kagawa, RM Hoffman and T Fujiwara. (2013). A genetically engineered oncolytic adenovirus decoys and lethally traps quiescent cancer stem-like cells in S/G2/M phases. Clin Cancer Res 19:6495-505.
76.Yang H, T Peng, J Li, Y Wang, W Zhang, P Zhang, S Peng, T Du, Y Li, Q Yan and B Liu. (2016). Treatment of colon cancer with oncolytic herpes simplex virus in preclinical models. Gene Ther 23:450-9.
77.Yoo SY, SY Bang, SN Jeong, DH Kang and J Heo. (2016). A cancer-favoring oncolytic vaccinia virus shows enhanced suppression of stem-cell like colon cancer. Oncotarget 7:16479-89.
78.Bach P, T Abel, C Hoffmann, Z Gal, G Braun, I Voelker, CR Ball, IC Johnston, UM Lauer, C Herold-Mende, MD Muhlebach, H Glimm and CJ Buchholz. (2013). Specific elimination of CD133+ tumor cells with targeted oncolytic measles virus. Cancer Res 73:865-74.
79.Thomas ED, S Meza-Perez, KS Bevis, TD Randall, GY Gillespie, C Langford and RD Alvarez. (2016). IL-12 Expressing oncolytic herpes simplex virus promotes anti-tumor activity and immunologic control of metastatic ovarian cancer in mice. J Ovarian Res 9:70.
80.(2017). First-Ever CAR T-cell Therapy Approved in U.S. Cancer Discov 7:OF1.
81.(2018). FDA Approves Second CAR T-cell Therapy. Cancer Discov 8:5-6.
82.Giavridis T, SJC van der Stegen, J Eyquem, M Hamieh, A Piersigilli and M Sadelain. (2018). CAR T cell-induced cytokine release syndrome is mediated by macrophages and abated by IL-1 blockade. Nat Med 24:731-738.
83.Hay KA. (2018). Cytokine release syndrome and neurotoxicity after CD19 chimeric antigen receptor-modified (CAR-) T cell therapy. Br J Haematol 183:364-374.
84.Norelli M, B Camisa, G Barbiera, L Falcone, A Purevdorj, M Genua, F Sanvito, M Ponzoni, C Doglioni, P Cristofori, C Traversari, C Bordignon, F Ciceri, R Ostuni, C Bonini, M Casucci and A Bondanza. (2018). Monocyte-derived IL-1 and IL-6 are differentially required for cytokine-release syndrome and neurotoxicity due to CAR T cells. Nat Med 24:739-748.
85.Shi J, TT Ma and HS Liu. (2018). [Research Progress on Dendritic Cells Combined with Cytokine Induced Killer Cells Immunotherapy for Hematological Malignancies –Review]. Zhongguo Shi Yan Xue Ye Xue Za Zhi 26:1235-1239.
86.Huang X, Y Li, L Shou, L Li, Z Chen, X Ye and W Qian. (2019). The molecular mechanisms underlying BCR/ABL degradation in chronic myeloid leukemia cells promoted by Beclin1-mediated autophagy. Cancer Manag Res 11:5197-5208.
87.Liu L, J Ma, L Qin, X Shi, H Si and Y Wei. (2019). Interleukin-24 enhancing antitumor activity of chimeric oncolytic adenovirus for treating acute promyelocytic leukemia cell. Medicine (Baltimore) 98:e15875.
88.Zhu HB, Q Deng, R Zhang, YY Jiang, JX Meng, MF Zhao, YM Li and R Cui. (2018). [Effect of PD-1 inhibitor Nivolumab on the proliferation and cytotoxicity of anti-CD19 chimeric antigen receptor T cells]. Zhonghua Xue Ye Xue Za Zhi 39:584-588.
89.Nakajima M, Y Sakoda, K Adachi, H Nagano and K Tamada. (2019). Improved survival of chimeric antigen receptor-engineered T (CAR-T) and tumor-specific T cells caused by anti-programmed cell death protein 1 single-chain variable fragment-producing CAR-T cells. Cancer Sci 110:3079-3088.
90.Zhu Y, X Hu, L Feng, Z Yang, L Zhou, X Duan, S Cheng, W Zhang, B Liu and K Zhang. (2019). Enhanced Therapeutic Efficacy of a Novel Oncolytic Herpes Simplex Virus Type 2 Encoding an Antibody Against Programmed Cell Death 1. Mol Ther Oncolytics 15:201-213.
91.Banerjee MN, R Bolli and JM Hare. (2018). Clinical Studies of Cell Therapy in Cardiovascular Medicine: Recent Developments and Future Directions. Circ Res 123:266-287.
92.Dominici M, K Le Blanc, I Mueller, I Slaper-Cortenbach, F Marini, D Krause, R Deans, A Keating, D Prockop and E Horwitz. (2006). Minimal criteria for defining multipotent mesenchymal stromal cells. The International Society for Cellular Therapy position statement. Cytotherapy 8:315-7.
93.Wagner W, F Wein, A Seckinger, M Frankhauser, U Wirkner, U Krause, J Blake, C Schwager, V Eckstein, W Ansorge and AD Ho. (2005). Comparative characteristics of mesenchymal stem cells from human bone marrow, adipose tissue, and umbilical cord blood. Exp Hematol 33:1402-16.
94.Mariotti E, P Mirabelli, G Abate, M Schiattarella, P Martinelli, G Fortunato, R Di Noto and L Del Vecchio. (2008). Comparative characteristics of mesenchymal stem cells from human bone marrow and placenta: CD10, CD49d, and CD56 make a difference. Stem Cells Dev 17:1039-41.
95.Joel MDM, J Yuan, J Wang, Y Yan, H Qian, X Zhang, W Xu and F Mao. (2019). MSC: immunoregulatory effects, roles on neutrophils and evolving clinical potentials. Am J Transl Res 11:3890-3904.
96.Uder C, S Bruckner, S Winkler, HM Tautenhahn and B Christ. (2018). Mammalian MSC from selected species: Features and applications. Cytometry A 93:32-49.
97.Oh KW, C Moon, HY Kim, SI Oh, J Park, JH Lee, IY Chang, KS Kim and SH Kim. (2015). Phase I trial of repeated intrathecal autologous bone marrow-derived mesenchymal stromal cells in amyotrophic lateral sclerosis. Stem Cells Transl Med 4:590-7.
98.Pezzanite LM, LA Fortier, DF Antczak, JM Cassano, MM Brosnahan, D Miller and LV Schnabel. (2015). Equine allogeneic bone marrow-derived mesenchymal stromal cells elicit antibody responses in vivo. Stem Cell Res Ther 6:54.
99.Staff NP, NN Madigan, J Morris, M Jentoft, EJ Sorenson, G Butler, D Gastineau, A Dietz and AJ Windebank. (2016). Safety of intrathecal autologous adipose-derived mesenchymal stromal cells in patients with ALS. Neurology 87:2230-2234.
100.Sykova E, P Rychmach, I Drahoradova, S Konradova, K Ruzickova, I Vorisek, S Forostyak, A Homola and M Bojar. (2017). Transplantation of Mesenchymal Stromal Cells in Patients With Amyotrophic Lateral Sclerosis: Results of Phase I/IIa Clinical Trial. Cell Transplant 26:647-658.
101.Connick P, M Kolappan, R Patani, MA Scott, C Crawley, XL He, K Richardson, K Barber, DJ Webber, CA Wheeler-Kingshott, DJ Tozer, RS Samson, DL Thomas, MQ Du, SL Luan, AW Michell, DR Altmann, AJ Thompson, DH Miller, A Compston and S Chandran. (2011). The mesenchymal stem cells in multiple sclerosis (MSCIMS) trial protocol and baseline cohort characteristics: an open-label pre-test: post-test study with blinded outcome assessments. Trials 12:62.
102.Connick P, M Kolappan, C Crawley, DJ Webber, R Patani, AW Michell, MQ Du, SL Luan, DR Altmann, AJ Thompson, A Compston, MA Scott, DH Miller and S Chandran. (2012). Autologous mesenchymal stem cells for the treatment of secondary progressive multiple sclerosis: an open-label phase 2a proof-of-concept study. Lancet Neurol 11:150-6.
103.Llufriu S, M Sepulveda, Y Blanco, P Marin, B Moreno, J Berenguer, I Gabilondo, E Martinez-Heras, N Sola-Valls, JA Arnaiz, EJ Andreu, B Fernandez, S Bullich, B Sanchez-Dalmau, F Graus, P Villoslada and A Saiz. (2014). Randomized placebo-controlled phase II trial of autologous mesenchymal stem cells in multiple sclerosis. PLoS One 9:e113936.
104.Karussis D, C Karageorgiou, A Vaknin-Dembinsky, B Gowda-Kurkalli, JM Gomori, I Kassis, JW Bulte, P Petrou, T Ben-Hur, O Abramsky and S Slavin. (2010). Safety and immunological effects of mesenchymal stem cell transplantation in patients with multiple sclerosis and amyotrophic lateral sclerosis. Arch Neurol 67:1187-94.
105.Ra JC, IS Shin, SH Kim, SK Kang, BC Kang, HY Lee, YJ Kim, JY Jo, EJ Yoon, HJ Choi and E Kwon. (2011). Safety of intravenous infusion of human adipose tissue-derived mesenchymal stem cells in animals and humans. Stem Cells Dev 20:1297-308.
106.Mendonca MV, TF Larocca, BS de Freitas Souza, CF Villarreal, LF Silva, AC Matos, MA Novaes, CM Bahia, AC de Oliveira Melo Martinez, CM Kaneto, SB Furtado, GP Sampaio, MB Soares and RR dos Santos. (2014). Safety and neurological assessments after autologous transplantation of bone marrow mesenchymal stem cells in subjects with chronic spinal cord injury. Stem Cell Res Ther 5:126.
107.Oh SK, KH Choi, JY Yoo, DY Kim, SJ Kim and SR Jeon. (2016). A Phase III Clinical Trial Showing Limited Efficacy of Autologous Mesenchymal Stem Cell Therapy for Spinal Cord Injury. Neurosurgery 78:436-47; discussion 447.
108.Jo CH, YG Lee, WH Shin, H Kim, JW Chai, EC Jeong, JE Kim, H Shim, JS Shin, IS Shin, JC Ra, S Oh and KS Yoon. (2014). Intra-articular injection of mesenchymal stem cells for the treatment of osteoarthritis of the knee: a proof-of-concept clinical trial. Stem Cells 32:1254-66.
109.Pers YM, L Rackwitz, R Ferreira, O Pullig, C Delfour, F Barry, L Sensebe, L Casteilla, S Fleury, P Bourin, D Noel, F Canovas, C Cyteval, G Lisignoli, J Schrauth, D Haddad, S Domergue, U Noeth, C Jorgensen and A Consortium. (2016). Adipose Mesenchymal Stromal Cell-Based Therapy for Severe Osteoarthritis of the Knee: A Phase I Dose-Escalation Trial. Stem Cells Transl Med 5:847-56.
110.Sanchez-Guijo F, T Caballero-Velazquez, O Lopez-Villar, A Redondo, R Parody, C Martinez, E Olavarria, E Andreu, F Prosper, M Diez-Campelo, C Regidor, E Villaron, L Lopez-Corral, D Caballero, MC Canizo and JA Perez-Simon. (2014). Sequential third-party mesenchymal stromal cell therapy for refractory acute graft-versus-host disease. Biol Blood Marrow Transplant 20:1580-5.
111.Ringden O, M Uzunel, I Rasmusson, M Remberger, B Sundberg, H Lonnies, HU Marschall, A Dlugosz, A Szakos, Z Hassan, B Omazic, J Aschan, L Barkholt and K Le Blanc. (2006). Mesenchymal stem cells for treatment of therapy-resistant graft-versus-host disease. Transplantation 81:1390-7.
112.Prasad VK, KG Lucas, GI Kleiner, JA Talano, D Jacobsohn, G Broadwater, R Monroy and J Kurtzberg. (2011). Efficacy and safety of ex vivo cultured adult human mesenchymal stem cells (Prochymal) in pediatric patients with severe refractory acute graft-versus-host disease in a compassionate use study. Biol Blood Marrow Transplant 17:534-41.
113.Dhere T, I Copland, M Garcia, KY Chiang, R Chinnadurai, M Prasad, J Galipeau and S Kugathasan. (2016). The safety of autologous and metabolically fit bone marrow mesenchymal stromal cells in medically refractory Crohn’s disease – a phase 1 trial with three doses. Aliment Pharmacol Ther 44:471-81.
114.Forbes GM, MJ Sturm, RW Leong, MP Sparrow, D Segarajasingam, AG Cummins, M Phillips and RP Herrmann. (2014). A phase 2 study of allogeneic mesenchymal stromal cells for luminal Crohn’s disease refractory to biologic therapy. Clin Gastroenterol Hepatol 12:64-71.
115.Soeder Y, M Loss, CL Johnson, JA Hutchinson, J Haarer, N Ahrens, R Offner, RJ Deans, G Van Bokkelen, EK Geissler, HJ Schlitt and MH Dahlke. (2015). First-in-Human Case Study: Multipotent Adult Progenitor Cells for Immunomodulation After Liver Transplantation. Stem Cells Transl Med 4:899-904.
116.Shi M, Z Zhang, R Xu, H Lin, J Fu, Z Zou, A Zhang, J Shi, L Chen, S Lv, W He, H Geng, L Jin, Z Liu and FS Wang. (2012). Human mesenchymal stem cell transfusion is safe and improves liver function in acute-on-chronic liver failure patients. Stem Cells Transl Med 1:725-31.
117.Reinders ME, JW de Fijter, H Roelofs, IM Bajema, DK de Vries, AF Schaapherder, FH Claas, PP van Miert, DL Roelen, C van Kooten, WE Fibbe and TJ Rabelink. (2013). Autologous bone marrow-derived mesenchymal stromal cells for the treatment of allograft rejection after renal transplantation: results of a phase I study. Stem Cells Transl Med 2:107-11.
118.Hare JM, JE Fishman, G Gerstenblith, DL DiFede Velazquez, JP Zambrano, VY Suncion, M Tracy, E Ghersin, PV Johnston, JA Brinker, E Breton, J Davis-Sproul, IH Schulman, J Byrnes, AM Mendizabal, MH Lowery, D Rouy, P Altman, C Wong Po Foo, P Ruiz, A Amador, J Da Silva, IK McNiece, AW Heldman, R George and A Lardo. (2012). Comparison of allogeneic vs autologous bone marrow-derived mesenchymal stem cells delivered by transendocardial injection in patients with ischemic cardiomyopathy: the POSEIDON randomized trial. JAMA 308:2369-79.
119.Lee JW, SH Lee, YJ Youn, MS Ahn, JY Kim, BS Yoo, J Yoon, W Kwon, IS Hong, K Lee, J Kwan, KS Park, D Choi, YS Jang and MK Hong. (2014). A randomized, open-label, multicenter trial for the safety and efficacy of adult mesenchymal stem cells after acute myocardial infarction. J Korean Med Sci 29:23-31.
120.Evans MJ and MH Kaufman. (1981). Establishment in culture of pluripotential cells from mouse embryos. Nature 292:154-6.
121.Martin GR. (1981). Isolation of a pluripotent cell line from early mouse embryos cultured in medium conditioned by teratocarcinoma stem cells. Proc Natl Acad Sci U S A 78:7634-8.
122.Thomson JA, J Itskovitz-Eldor, SS Shapiro, MA Waknitz, JJ Swiergiel, VS Marshall and JM Jones. (1998). Embryonic stem cell lines derived from human blastocysts. Science 282:1145-7.
123.Takahashi K and S Yamanaka. (2006). Induction of pluripotent stem cells from mouse embryonic and adult fibroblast cultures by defined factors. Cell 126:663-76.
124.Yu J, MA Vodyanik, K Smuga-Otto, J Antosiewicz-Bourget, JL Frane, S Tian, J Nie, GA Jonsdottir, V Ruotti, R Stewart, Slukvin, II and JA Thomson. (2007). Induced pluripotent stem cell lines derived from human somatic cells. Science 318:1917-20.
125.Ben-David U and N Benvenisty. (2011). The tumorigenicity of human embryonic and induced pluripotent stem cells. Nat Rev Cancer 11:268-77.
126.Ambasudhan R, N Dolatabadi, A Nutter, E Masliah, SR McKercher and SA Lipton. (2014). Potential for cell therapy in Parkinson’s disease using genetically programmed human embryonic stem cell-derived neural progenitor cells. J Comp Neurol 522:2845-56.
127.Zhu K, Q Wu, C Ni, P Zhang, Z Zhong, Y Wu, Y Wang, Y Xu, M Kong, H Cheng, Z Tao, Q Yang, H Liang, Y Jiang, Q Li, J Zhao, J Huang, F Zhang, Q Chen, Y Li, J Chen, W Zhu, H Yu, J Zhang, H-T Yang, X Hu and Ja Wang. (2018). Lack of Remuscularization Following Transplantation of Human Embryonic Stem Cell-Derived Cardiovascular Progenitor Cells in Infarcted Nonhuman Primates. Circulation Research.
128.Caspi O, I Huber, I Kehat, M Habib, G Arbel, A Gepstein, L Yankelson, D Aronson, R Beyar and L Gepstein. (2007). Transplantation of human embryonic stem cell-derived cardiomyocytes improves myocardial performance in infarcted rat hearts. J Am Coll Cardiol 50:1884-93.
129.Chong JJ, X Yang, CW Don, E Minami, YW Liu, JJ Weyers, WM Mahoney, B Van Biber, SM Cook, NJ Palpant, JA Gantz, JA Fugate, V Muskheli, GM Gough, KW Vogel, CA Astley, CE Hotchkiss, A Baldessari, L Pabon, H Reinecke, EA Gill, V Nelson, HP Kiem, MA Laflamme and CE Murry. (2014). Human embryonic-stem-cell-derived cardiomyocytes regenerate non-human primate hearts. Nature 510:273-7.
130.Li Z, KD Wilson, B Smith, DL Kraft, F Jia, M Huang, X Xie, RC Robbins, SS Gambhir, IL Weissman and JC Wu. (2009). Functional and transcriptional characterization of human embryonic stem cell-derived endothelial cells for treatment of myocardial infarction. PLoS One 4:e8443.
131.Cheung C and S Sinha. (2011). Human embryonic stem cell-derived vascular smooth muscle cells in therapeutic neovascularisation. J Mol Cell Cardiol 51:651-64.
132.Schwartz SD, CD Regillo, BL Lam, D Eliott, PJ Rosenfeld, NZ Gregori, JP Hubschman, JL Davis, G Heilwell, M Spirn, J Maguire, R Gay, J Bateman, RM Ostrick, D Morris, M Vincent, E Anglade, LV Del Priore and R Lanza. (2015). Human embryonic stem cell-derived retinal pigment epithelium in patients with age-related macular degeneration and Stargardt’s macular dystrophy: follow-up of two open-label phase 1/2 studies. Lancet 385:509-16.
133.Robinton DA and GQ Daley. (2012). The promise of induced pluripotent stem cells in research and therapy. Nature 481:295-305.
134.Bolli R, AR Chugh, D D’Amario, JH Loughran, MF Stoddard, S Ikram, GM Beache, SG Wagner, A Leri, T Hosoda, F Sanada, JB Elmore, P Goichberg, D Cappetta, NK Solankhi, I Fahsah, DG Rokosh, MS Slaughter, J Kajstura and P Anversa. (2011). Cardiac stem cells in patients with ischaemic cardiomyopathy (SCIPIO): initial results of a randomised phase 1 trial. Lancet 378:1847-57.
135.Makkar RR, RR Smith, K Cheng, K Malliaras, LE Thomson, D Berman, LS Czer, L Marban, A Mendizabal, PV Johnston, SD Russell, KH Schuleri, AC Lardo, G Gerstenblith and E Marban. (2012). Intracoronary cardiosphere-derived cells for heart regeneration after myocardial infarction (CADUCEUS): a prospective, randomised phase 1 trial. Lancet 379:895-904.
136.Bartunek J, A Terzic, BA Davison, GS Filippatos, S Radovanovic, B Beleslin, B Merkely, P Musialek, W Wojakowski, P Andreka, IG Horvath, A Katz, D Dolatabadi, B El Nakadi, A Arandjelovic, I Edes, PM Seferovic, S Obradovic, M Vanderheyden, N Jagic, I Petrov, S Atar, M Halabi, VL Gelev, MK Shochat, JD Kasprzak, R Sanz-Ruiz, GR Heyndrickx, N Nyolczas, V Legrand, A Guedes, A Heyse, T Moccetti, F Fernandez-Aviles, P Jimenez-Quevedo, A Bayes-Genis, JM Hernandez-Garcia, F Ribichini, M Gruchala, SA Waldman, JR Teerlink, BJ Gersh, TJ Povsic, TD Henry, M Metra, RJ Hajjar, M Tendera, A Behfar, B Alexandre, A Seron, WG Stough, W Sherman, G Cotter, W Wijns and C Program. (2017). Cardiopoietic cell therapy for advanced ischaemic heart failure: results at 39 weeks of the prospective, randomized, double blind, sham-controlled CHART-1 clinical trial. Eur Heart J 38:648-660.
137.Vrtovec B, G Poglajen, L Lezaic, M Sever, D Domanovic, P Cernelc, A Socan, S Schrepfer, G Torre-Amione, F Haddad and JC Wu. (2013). Effects of intracoronary CD34+ stem cell transplantation in nonischemic dilated cardiomyopathy patients: 5-year follow-up. Circ Res 112:165-73.
138.Vrtovec B, G Poglajen, M Sever, L Lezaic, A Socan, F Haddad and JC Wu. (2013). CD34+ stem cell therapy in nonischemic dilated cardiomyopathy patients. Clin Pharmacol Ther 94:452-8.
139.Riley J, J Glass, EL Feldman, M Polak, J Bordeau, T Federici, K Johe and NM Boulis. (2014). Intraspinal stem cell transplantation in amyotrophic lateral sclerosis: a phase I trial, cervical microinjection, and final surgical safety outcomes. Neurosurgery 74:77-87.
140.Feldman EL, NM Boulis, J Hur, K Johe, SB Rutkove, T Federici, M Polak, J Bordeau, SA Sakowski and JD Glass. (2014). Intraspinal neural stem cell transplantation in amyotrophic lateral sclerosis: phase 1 trial outcomes. Ann Neurol 75:363-73.
141.Mazzini L, M Gelati, DC Profico, G Soraru, D Ferrari, M Copetti, G Muzi, C Ricciolini, S Carletti, C Giorgi, C Spera, D Frondizi, S Masiero, A Stecco, C Cisari, E Bersano, F De Marchi, MF Sarnelli, G Querin, R Cantello, F Petruzzelli, A Maglione, C Zalfa, E Binda, A Visioli, D Trombetta, B Torres, L Bernardini, A Gaiani, M Massara, S Paolucci, NM Boulis, AL Vescovi and AL-NTS Group. (2019). Results from Phase I Clinical Trial with Intraspinal Injection of Neural Stem Cells in Amyotrophic Lateral Sclerosis: A Long-Term Outcome. Stem Cells Transl Med 8:887-897.
142.Garitaonandia I, R Gonzalez, T Christiansen-Weber, T Abramihina, M Poustovoitov, A Noskov, G Sherman, A Semechkin, E Snyder and R Kern. (2016). Neural Stem Cell Tumorigenicity and Biodistribution Assessment for Phase I Clinical Trial in Parkinson’s Disease. Sci Rep 6:34478.
143.Kalladka D, J Sinden, K Pollock, C Haig, J McLean, W Smith, A McConnachie, C Santosh, PM Bath, L Dunn and KW Muir. (2016). Human neural stem cells in patients with chronic ischaemic stroke (PISCES): a phase 1, first-in-man study. Lancet 388:787-96.
144.Karantalis V, VY Suncion-Loescher, L Bagno, S Golpanian, A Wolf, C Sanina, C Premer, AJ Kanelidis, F McCall, B Wang, W Balkan, J Rodriguez, M Rosado, A Morales, K Hatzistergos, M Natsumeda, I Margitich, IH Schulman, SA Gomes, M Mushtaq, DL DiFede, JE Fishman, P Pattany, JP Zambrano, AW Heldman and JM Hare. (2015). Synergistic Effects of Combined Cell Therapy for Chronic Ischemic Cardiomyopathy. J Am Coll Cardiol 66:1990-1999.
145.Natsumeda M, V Florea, AC Rieger, BA Tompkins, MN Banerjee, S Golpanian, J Fritsch, AM Landin, ND Kashikar, V Karantalis, VY Loescher, KE Hatzistergos, L Bagno, C Sanina, M Mushtaq, J Rodriguez, M Rosado, A Wolf, K Collon, L Vincent, AJ Kanelidis, IH Schulman, R Mitrani, AW Heldman, W Balkan and JM Hare. (2017). A Combination of Allogeneic Stem Cells Promotes Cardiac Regeneration. J Am Coll Cardiol 70:2504-2515.
146.Ye L, YH Chang, Q Xiong, P Zhang, L Zhang, P Somasundaram, M Lepley, C Swingen, L Su, JS Wendel, J Guo, A Jang, D Rosenbush, L Greder, JR Dutton, J Zhang, TJ Kamp, DS Kaufman, Y Ge and J Zhang. (2014). Cardiac repair in a porcine model of acute myocardial infarction with human induced pluripotent stem cell-derived cardiovascular cells. Cell Stem Cell 15:750-61.
147.Jansen K, CCL Schuurmans, J Jansen, R Masereeuw and T Vermonden. (2017). Hydrogel-Based Cell Therapies for Kidney Regeneration: Current Trends in Biofabrication and In Vivo Repair. Curr Pharm Des 23:3845-3857.
148.Nommiste B, K Fynes, VE Tovell, C Ramsden, L da Cruz and P Coffey. (2017). Stem cell-derived retinal pigment epithelium transplantation for treatment of retinal disease. Prog Brain Res 231:225-244.
149.Mollanoori H and S Teimourian. (2018). Therapeutic applications of CRISPR/Cas9 system in gene therapy. Biotechnol Lett 40:907-914.
150.Cai B, S Sun, Z Li, X Zhang, Y Ke, J Yang and X Li. (2018). Application of CRISPR/Cas9 technologies combined with iPSCs in the study and treatment of retinal degenerative diseases. Hum Genet 137:679-688.
151.Wang X, A Raghavan, T Chen, L Qiao, Y Zhang, Q Ding and K Musunuru. (2016). CRISPR-Cas9 Targeting of PCSK9 in Human Hepatocytes In Vivo-Brief Report. Arterioscler Thromb Vasc Biol 36:783-6.
152.Xie C, YP Zhang, L Song, J Luo, W Qi, J Hu, D Lu, Z Yang, J Zhang, J Xiao, B Zhou, JL Du, N Jing, Y Liu, Y Wang, BL Li, BL Song and Y Yan. (2016). Genome editing with CRISPR/Cas9 in postnatal mice corrects PRKAG2 cardiac syndrome. Cell Res 26:1099-1111.
153.Ma H, N Marti-Gutierrez, SW Park, J Wu, Y Lee, K Suzuki, A Koski, D Ji, T Hayama, R Ahmed, H Darby, C Van Dyken, Y Li, E Kang, AR Park, D Kim, ST Kim, J Gong, Y Gu, X Xu, D Battaglia, SA Krieg, DM Lee, DH Wu, DP Wolf, SB Heitner, JCI Belmonte, P Amato, JS Kim, S Kaul and S Mitalipov. (2017). Correction of a pathogenic gene mutation in human embryos. Nature 548:413-419.
154.Liu Y, Y Yang, X Kang, B Lin, Q Yu, B Song, G Gao, Y Chen, X Sun, X Li, L Bu and Y Fan. (2017). One-Step Biallelic and Scarless Correction of a beta-Thalassemia Mutation in Patient-Specific iPSCs without Drug Selection. Mol Ther Nucleic Acids 6:57-67.
155.Chang CW, YS Lai, E Westin, A Khodadadi-Jamayran, KM Pawlik, LS Lamb, Jr., FD Goldman and TM Townes. (2015). Modeling Human Severe Combined Immunodeficiency and Correction by CRISPR/Cas9-Enhanced Gene Targeting. Cell Rep 12:1668-77.
156.Stilhano RS, L Martins, SJM Ingham, JB Pesquero and J Huard. (2015). Gene and cell therapy for muscle regeneration. Curr Rev Musculoskelet Med 8:182-187.
157.Rund D and E Rachmilewitz. (2005). Beta-thalassemia. N Engl J Med 353:1135-46.
158.Cappellini MD, M Bejaoui, L Agaoglu, D Canatan, M Capra, A Cohen, G Drelichman, M Economou, S Fattoum, A Kattamis, Y Kilinc, S Perrotta, A Piga, JB Porter, L Griffel, V Dong, J Clark and Y Aydinok. (2011). Iron chelation with deferasirox in adult and pediatric patients with thalassemia major: efficacy and safety during 5 years’ follow-up. Blood 118:884-93.
159.Lucarelli G and J Gaziev. (2008). Advances in the allogeneic transplantation for thalassemia. Blood Rev 22:53-63.
160.Dong AC and S Rivella. (2017). Gene Addition Strategies for beta-Thalassemia and Sickle Cell Anemia. Adv Exp Med Biol 1013:155-176.
161.Thompson AA, MC Walters, J Kwiatkowski, JEJ Rasko, JA Ribeil, S Hongeng, E Magrin, GJ Schiller, E Payen, M Semeraro, D Moshous, F Lefrere, H Puy, P Bourget, A Magnani, L Caccavelli, JS Diana, F Suarez, F Monpoux, V Brousse, C Poirot, C Brouzes, JF Meritet, C Pondarre, Y Beuzard, S Chretien, T Lefebvre, DT Teachey, U Anurathapan, PJ Ho, C von Kalle, M Kletzel, E Vichinsky, S Soni, G Veres, O Negre, RW Ross, D Davidson, A Petrusich, L Sandler, M Asmal, O Hermine, M De Montalembert, S Hacein-Bey-Abina, S Blanche, P Leboulch and M Cavazzana. (2018). Gene Therapy in Patients with Transfusion-Dependent beta-Thalassemia. N Engl J Med 378:1479-1493.
162.Kohn DB and HB Gaspar. (2017). How We Manage Adenosine Deaminase-Deficient Severe Combined Immune Deficiency (ADA SCID). J Clin Immunol 37:351-356.
163.Ferrua F and A Aiuti. (2017). Twenty-Five Years of Gene Therapy for ADA-SCID: From Bubble Babies to an Approved Drug. Hum Gene Ther 28:972-981.
164.Bradford KL, FA Moretti, DA Carbonaro-Sarracino, HB Gaspar and DB Kohn. (2017). Adenosine Deaminase (ADA)-Deficient Severe Combined Immune Deficiency (SCID): Molecular Pathogenesis and Clinical Manifestations. J Clin Immunol 37:626-637.
165.Polmar SH, RC Stern, AL Schwartz, EM Wetzler, PA Chase and R Hirschhorn. (1976). Enzyme replacement therapy for adenosine deaminase deficiency and severe combined immunodeficiency. N Engl J Med 295:1337-43.
166.Hershfield MS, RH Buckley, ML Greenberg, AL Melton, R Schiff, C Hatem, J Kurtzberg, ML Markert, RH Kobayashi, AL Kobayashi and et al. (1987). Treatment of adenosine deaminase deficiency with polyethylene glycol-modified adenosine deaminase. N Engl J Med 316:589-96.
167.Cicalese MP, F Ferrua, L Castagnaro, R Pajno, F Barzaghi, S Giannelli, F Dionisio, I Brigida, M Bonopane, M Casiraghi, A Tabucchi, F Carlucci, E Grunebaum, M Adeli, RG Bredius, JM Puck, P Stepensky, I Tezcan, K Rolfe, E De Boever, RR Reinhardt, J Appleby, F Ciceri, MG Roncarolo and A Aiuti. (2016). Update on the safety and efficacy of retroviral gene therapy for immunodeficiency due to adenosine deaminase deficiency. Blood 128:45-54.
168.Gaspar HB, S Cooray, KC Gilmour, KL Parsley, F Zhang, S Adams, E Bjorkegren, J Bayford, L Brown, EG Davies, P Veys, L Fairbanks, V Bordon, T Petropoulou, C Kinnon and AJ Thrasher. (2011). Hematopoietic stem cell gene therapy for adenosine deaminase-deficient severe combined immunodeficiency leads to long-term immunological recovery and metabolic correction. Sci Transl Med 3:97ra80.
169.Santilli G, SI Thornhill, C Kinnon and AJ Thrasher. (2008). Gene therapy of inherited immunodeficiencies. Expert Opin Biol Ther 8:397-407.
170.Aiuti A, S Slavin, M Aker, F Ficara, S Deola, A Mortellaro, S Morecki, G Andolfi, A Tabucchi, F Carlucci, E Marinello, F Cattaneo, S Vai, P Servida, R Miniero, MG Roncarolo and C Bordignon. (2002). Correction of ADA-SCID by stem cell gene therapy combined with nonmyeloablative conditioning. Science 296:2410-3.
171.De Luca M, A Aiuti, G Cossu, M Parmar, G Pellegrini and PG Robey. (2019). Advances in stem cell research and therapeutic development. Nat Cell Biol 21:801-811.